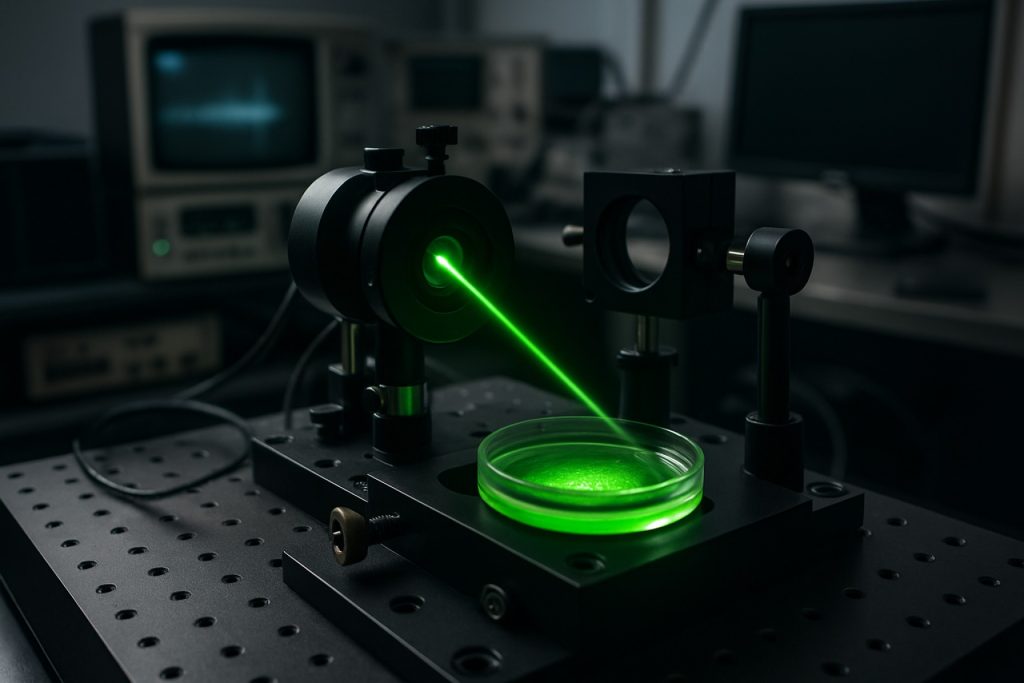
Table of Contents
- Executive Summary: Key Insights & 2025 Outlook
- Quantum Photobiology: Core Principles & Industry Applications
- 2025 Market Size and Growth Forecasts (2025–2030)
- Leading Manufacturers & Industry Alliances
- Breakthrough Technologies in Quantum Photobiology Equipment
- Regulatory Landscape & Standards (2025 Update)
- Emerging Use Cases: Medicine, Agriculture, and Beyond
- Competitive Landscape & Innovation Hotspots
- Supply Chain and Manufacturing Challenges
- Future Trends & Strategic Opportunities (2025–2030)
- Sources & References
Executive Summary: Key Insights & 2025 Outlook
The quantum photobiology equipment manufacturing sector is positioned for significant transformation and growth in 2025, fueled by advances in quantum optics, photonic engineering, and life sciences integration. This convergence is enabling the precise manipulation of light at the quantum level to investigate and influence biological processes, opening new frontiers in medical diagnostics, agricultural optimization, and phototherapy. Leading manufacturers are scaling up their investments in quantum light sources, photonic integrated circuits, and high-precision optical components to meet rising demand from both research and commercial end-users.
In 2025, major equipment manufacturers are accelerating the integration of quantum light emitting diodes (QLEDs), single-photon sources, and entangled photon generators into modular platforms for quantum photobiology applications. Companies such as Hamamatsu Photonics and Thorlabs are expanding their production capacity for high-sensitivity photodetectors and quantum-grade lasers, while Edmund Optics is focusing on quantum-optimized optical components, including beam splitters and interference filters tailored for biological sample analysis.
The sector’s innovation pipeline is further bolstered by strategic partnerships between photonics firms and biotechnology players, aiming to co-develop turnkey systems for quantum-assisted cellular imaging and real-time molecular sensing. For example, collaborative efforts are underway to deliver compact quantum fluorescence microscopes and portable quantum spectrometers, targeting both laboratory and field applications. This is complemented by increased investment in automation, with manufacturers incorporating AI-driven calibration and quality control systems to enhance throughput, reliability, and reproducibility.
The global landscape is witnessing robust regional activity, particularly in North America, Europe, and East Asia, where government-backed quantum initiatives and public-private consortia are providing incentives for advanced manufacturing. Supply chain resilience is a key priority, with firms diversifying sourcing strategies for key photonic crystals, quantum dots, and semiconductor substrates.
Looking ahead, the quantum photobiology equipment manufacturing industry is expected to achieve double-digit annual growth rates through 2027, propelled by expanding application domains in precision agriculture, regenerative medicine, and pharmaceutical R&D. The rapid pace of technical standardization and regulatory engagement is anticipated to further accelerate market adoption. As new quantum photobiology modalities transition from research prototypes to scalable products, manufacturers are poised to capture opportunities across both developed and emerging markets, reinforcing the industry’s trajectory as a critical enabler of next-generation biotechnological innovation.
Quantum Photobiology: Core Principles & Industry Applications
Quantum photobiology equipment manufacturing is transitioning from niche laboratory production to a more scalable, semi-commercial phase as of 2025. This shift is catalyzed by rising demand for precision measurement devices and experimental platforms that exploit quantum effects to probe biological systems—such as single-photon detectors, quantum light sources, and spectroscopic tools. The field is primarily underpinned by advances in quantum optics, semiconductor nanofabrication, and integration of photon management technologies, allowing researchers to interrogate biological phenomena at previously inaccessible resolutions.
A current hallmark of activity is the close collaboration between photonics manufacturers and quantum technology developers. For example, Hamamatsu Photonics is recognized for its cutting-edge photomultiplier tubes and single-photon counting modules, foundational for quantum-level bio-imaging and spectroscopy. These are increasingly tailored for biophotonic applications, with new iterations offering improved sensitivity and reduced background noise essential for quantum photobiology experiments. Similarly, Thorlabs continues to expand its portfolio of quantum light sources, optical components, and cryogenic platforms, enabling custom assembly of experimental setups for research institutions and emerging biotech start-ups.
On the supply side, semiconductor and nanofabrication specialists like ams OSRAM are leveraging their vertical integration to supply quantum dot-based emitters and detectors adapted for biological sample compatibility. These components are crucial for both commercial and research-grade quantum photobiology instruments, and manufacturers are investing in production lines that meet cleanroom and ISO standards required for bioanalytical applications. Companies such as Carl Zeiss AG have begun integrating quantum-enhanced detection modules into advanced microscopy systems, signaling an anticipated convergence of quantum detection with mainstream biomedical imaging platforms.
Looking ahead to the next few years, the trajectory of quantum photobiology equipment manufacturing is expected to hinge on two major trends: miniaturization and system integration. Industry consortia are forming to standardize interfaces and component interoperability, which will accelerate the development of plug-and-play modules for both academic and clinical settings. Furthermore, public-private partnerships with leading national laboratories and quantum technology institutes are likely to foster pilot production lines for scalable quantum photobiology tools.
Although large-scale commercialization is still nascent, the maturation of manufacturing ecosystems around quantum photobiology is poised to drive down costs, enhance device performance, and open new market opportunities across fundamental research, drug discovery, and diagnostics by 2027 and beyond.
2025 Market Size and Growth Forecasts (2025–2030)
The market for quantum photobiology equipment manufacturing is poised for significant development in 2025 and the following years, propelled by advances in quantum technologies, photonics, and a growing demand for innovative biotechnological solutions. Quantum photobiology equipment, which leverages quantum properties of light for biological research and applications, is transitioning from niche academic use to broader adoption in pharmaceutical, agricultural, and medical sectors. This transition is supported by the increasing integration of quantum-based light sources, such as single-photon emitters and entangled photon systems, into photobiology instrumentation.
Industry leaders in quantum and photonics equipment, including Thorlabs, Inc., Hamamatsu Photonics K.K., and Carl Zeiss AG, have amplified research and development investments to address expanding commercial interest. In 2025, these companies are expected to introduce next-generation equipment featuring enhanced wavelength specificity, quantum coherence controls, and improved detection capabilities, targeting applications in quantum imaging, biosensing, and photodynamic therapy. For instance, Hamamatsu Photonics K.K. has announced the development of quantum dot-based imaging systems, and Carl Zeiss AG is increasing its focus on quantum-enhanced microscopy platforms.
Market expansion is further supported by government and institutional funding initiatives in the United States, Europe, and Asia, emphasizing quantum technology commercialization and life sciences innovation. In 2025, collaborations between industrial manufacturers and prominent research institutions are expected to accelerate prototyping and deployment. The presence of dedicated manufacturing clusters and the entry of new players—particularly startups formed as spinouts from university quantum photonics labs—are projected to increase competition and diversify product offerings.
Forecasts for 2025 to 2030 suggest a compound annual growth rate (CAGR) exceeding 20% for the quantum photobiology equipment sector, outpacing traditional photobiology equipment markets. This growth is driven by anticipated breakthroughs in quantum light source miniaturization, improved system stability, and growing end-user acceptance in life sciences and healthcare. The Asia-Pacific region, led by increased manufacturing capacities in Japan and China, is expected to emerge as a primary production and export hub, while North America and Europe continue to drive high-value R&D and system integration.
Nevertheless, the sector faces challenges related to the scalability of quantum devices, component standardization, and the need for specialized technical expertise. To address these, leading manufacturers such as Thorlabs, Inc. and Hamamatsu Photonics K.K. are investing in training programs and collaborative industry standards initiatives, aiming to support sustainable growth and rapid technology adoption through 2030.
Leading Manufacturers & Industry Alliances
As of 2025, the quantum photobiology equipment manufacturing sector is characterized by a dynamic ecosystem of established leaders, emerging innovators, and strategic industry alliances. The field is driven by rapid advancements in quantum optics, photonics, and life sciences integration, with equipment designed for applications ranging from advanced imaging to precision-driven phototherapy.
Among the leading manufacturers, Thorlabs continues to play a pivotal role. Renowned for its broad photonics portfolio, Thorlabs has expanded its manufacturing capabilities to produce quantum photobiology instruments, such as single-photon detectors and tunable laser sources, which are essential for experimental and applied quantum biology. Similarly, Hamamatsu Photonics is a key supplier of high-sensitivity photodetectors and light sources, supporting both OEMs and research institutions in quantum-enabled biological analysis.
Another significant player is Carl Zeiss AG, whose precision optics and microscopy solutions are being adapted for quantum photobiology workflows. Zeiss’s collaborations with leading academic and industrial partners have facilitated the integration of quantum light sources into next-generation imaging platforms. In parallel, Oxford Instruments has made notable strides in quantum sensor manufacturing, with their quantum photonics division focusing on scalable production for life science applications.
Industry alliances and consortia are increasingly important for standardization, knowledge exchange, and technology transfer. The European Photonics Industry Consortium (EPIC) has established dedicated working groups focused on quantum photonics and biophotonics convergence, fostering collaboration between component suppliers, systems integrators, and end-users. Additionally, Photonics21 is promoting cross-sector innovation projects funded under the EU’s Horizon Europe program, supporting joint ventures and pilot manufacturing lines for quantum photobiology instrumentation.
Looking ahead, the sector is expected to see further consolidation of supply chains and increased investment in scalable manufacturing. Multiple manufacturers are exploring vertical integration, combining quantum device fabrication with system-level assembly to streamline performance and reliability. As quantum photobiology moves from research toward clinical and industrial deployment, alliances between equipment manufacturers and biotechnology firms are likely to accelerate, driving both technological maturity and regulatory alignment over the next few years.
Breakthrough Technologies in Quantum Photobiology Equipment
Quantum photobiology equipment manufacturing is experiencing a period of rapid technological evolution in 2025, driven by advances in quantum optics, nanophotonics, and integrated photonic circuits. One of the most significant breakthroughs centers on the integration of quantum dots and single-photon emitters into photobiological instrumentation, allowing for unprecedented sensitivity and spatial resolution in probing biological systems. Manufacturers are leveraging these innovations to design devices capable of detecting minute photonic interactions, essential for studying quantum effects in photosynthetic complexes and neural phototransduction.
A notable trend is the adoption of on-chip quantum light sources within commercial spectrometers and imaging platforms. These chips, often based on silicon photonics, enable scalable and repeatable production, reducing the costs and complexity of quantum-grade equipment. Companies such as IBM and Carl Zeiss AG have been at the forefront, showcasing prototype systems in 2024 that incorporate quantum optical components for life sciences applications. These systems offer enhanced detection of photon-based signals at the single-molecule level, opening new avenues for research into energy transfer in biomolecules and light-driven cellular processes.
Another breakthrough is the emergence of hybrid quantum-classical measurement systems that combine the statistical power of quantum sensing with robust classical data acquisition. This approach is being actively developed by Thorlabs, which has introduced modular platforms compatible with quantum detectors and conventional photonic instrumentation. Such hybridization is crucial for translating laboratory-scale quantum photobiology experiments into reproducible, high-throughput industrial and clinical workflows.
Looking to 2025 and the near future, the manufacturing sector is also witnessing increased collaboration with academic and governmental research bodies, fostering open standards for quantum photonic interfaces and calibration protocols. This is exemplified by initiatives from Carl Zeiss AG and IBM in partnership with European quantum technology consortia, aiming to ensure interoperability and scalability as quantum photobiology matures from prototype devices to commercial deployment.
Overall, the sector’s outlook is marked by optimism, with rapid iteration cycles expected to continue through the next few years. The convergence of quantum-grade photonic manufacturing, scalable integration, and hybrid measurement architectures is anticipated to accelerate the adoption of quantum photobiology equipment in both research and applied clinical settings.
Regulatory Landscape & Standards (2025 Update)
The regulatory landscape and standards governing quantum photobiology equipment manufacturing are evolving rapidly in 2025, reflecting the sector’s transition from experimental research to early-stage commercialization. As quantum photobiology devices—leveraging quantum effects to manipulate biological processes with light—move closer to market, regulators and standards bodies are working to ensure safety, efficacy, and interoperability.
Currently, most regulations impacting quantum photobiology equipment fall under broader frameworks that address medical devices, lasers, and photonic instrumentation. For example, in the United States, the U.S. Food and Drug Administration (FDA) continues to require premarket clearance or approval for devices intended for medical use, with quantum-enabled systems generally evaluated under existing device codes for phototherapy or diagnostic imaging. The FDA is in the process of reviewing whether specialized guidance is needed as quantum photobiology technologies begin clinical trials and early deployments.
Similarly, in Europe, the European Medicines Agency (EMA) and national competent authorities apply the Medical Device Regulation (MDR) to quantum photobiology equipment, but several working groups within the CEN-CENELEC standards committees are initiating new work items to address quantum-specific risk factors, such as novel quantum light sources and entanglement-based measurement protocols.
Internationally, the International Organization for Standardization (ISO) is gathering input through technical committees such as ISO/TC 126 (Photonics) and ISO/TC 229 (Nanotechnologies) on the need for harmonized standards related to photobiological safety, quantum light calibration, and device interoperability. The emergence of quantum photobiology testbeds—led by consortia that include manufacturers like Hamamatsu Photonics—is providing real-world data that informs these standardization efforts.
Looking ahead, the next few years are expected to see the publication of draft technical standards specifically addressing quantum photobiology, including protocols for quantum light dosimetry and bio-effect validation. Regulatory agencies are likely to increase engagement with equipment manufacturers to align standards with evolving industrial capabilities. The progress of these initiatives will shape the pace of clinical adoption and international trade in quantum photobiology equipment, while ongoing collaboration between bodies such as ISO, FDA, and industry leaders will be crucial for global harmonization.
Emerging Use Cases: Medicine, Agriculture, and Beyond
Quantum photobiology equipment manufacturing is rapidly transitioning from foundational research to early-stage commercialization, with significant implications across medicine, agriculture, and other sectors. In 2025, a growing number of companies are developing and refining precision instruments that exploit quantum effects in light-matter interactions, enabling unprecedented control over biological processes. This new generation of equipment includes quantum-enhanced imaging systems, ultra-sensitive biosensors, and programmable photonic devices designed for cellular manipulation.
In medicine, quantum photobiology equipment is enabling non-invasive diagnostics and targeted therapies. Companies are introducing imaging systems that utilize quantum entanglement and single-photon detection to achieve super-resolution visualization of cellular structures, facilitating early disease detection and characterization at the molecular level. For example, quantum-based fluorescence microscopes and photonic biosensors are now being piloted in clinical settings to improve the accuracy of cancer diagnostics and monitor real-time cellular responses to drug treatments. Leading photonics manufacturers are expanding their portfolios to include quantum-enabled devices, with several partnerships announced in 2024 and 2025 to co-develop specialized medical imaging solutions (Hamamatsu Photonics).
Agricultural applications are also emerging, as quantum photobiology tools allow for precise manipulation of photosynthetic pathways and plant growth cycles. Equipment manufacturers are collaborating with agri-tech firms to develop quantum light modulators and spectrum-tuning systems that can optimize crop yields and enhance resistance to environmental stressors. These systems leverage quantum-level control of light quality and intensity, enabling tailored growth environments for high-value crops in controlled-environment agriculture (CEA) facilities. Major players in optoelectronics and industrial lighting are investing in quantum technology R&D to address the growing demand for sustainable, high-efficiency agricultural production (OSRAM).
Beyond medicine and agriculture, quantum photobiology equipment is being explored for applications in environmental monitoring, biosecurity, and advanced materials research. Ultra-sensitive quantum biosensors are under development for detecting pathogens, toxins, and pollutants at extremely low concentrations, offering new capabilities for public health and environmental safety. Furthermore, quantum-enhanced biophotonic platforms are facilitating novel research into biomolecular quantum effects, opening pathways to next-generation materials and energy conversion systems.
Looking ahead, the outlook for quantum photobiology equipment manufacturing is robust, with market entry barriers gradually lowering as enabling photonic and quantum technologies mature. Industry alliances and government-backed innovation programs are accelerating commercialization, while ongoing advances in miniaturization and integration bode well for wider adoption in the next few years. As quantum photobiology transitions from research labs to practical deployments, the sector is poised to deliver transformative benefits across multiple domains.
Competitive Landscape & Innovation Hotspots
The competitive landscape of quantum photobiology equipment manufacturing in 2025 is marked by a convergence of photonics, quantum technologies, and advanced biology instrumentation. Several established photonics and quantum technology companies are entering or expanding their offerings in this nascent field, while specialized start-ups and academic spin-offs are pushing the boundaries of innovation. This sector is characterized by rapid prototyping, collaborative R&D, and the integration of quantum light sources, single-photon detectors, and precision bio-optical interfaces.
Key players include leading quantum optics manufacturers such as Thorlabs and Hamamatsu Photonics, both of which have introduced highly sensitive photon counting modules and quantum light sources that are adaptable for advanced photobiology research. Carl Zeiss AG and Olympus Corporation are also investing in quantum-enhanced microscopy systems, aiming to provide life science researchers with unprecedented spatial and temporal resolution in live cell imaging.
A prominent innovation hotspot lies in the integration of quantum dot light sources and single-photon avalanche diodes (SPADs) for ultra-sensitive detection of biophotonic signals. Companies like Excelitas Technologies are actively developing next-generation SPAD arrays and time-correlated single photon counting (TCSPC) modules, which are vital for time-resolved fluorescence and photon counting in quantum photobiology applications. Meanwhile, ID Quantique is leveraging its expertise in quantum photonics to design devices capable of manipulating and detecting quantum states of light for biological assays with single-molecule sensitivity.
Industry collaborations are increasingly common, with manufacturers partnering with university research centers and national laboratories to accelerate the commercialization of quantum photobiology tools. For example, quantum-enabled super-resolution microscopy and entangled photon-based spectroscopies are emerging as key application areas, supported by initiatives from photonics consortia and standardization bodies.
Looking ahead to the next few years, the sector is expected to see intensified competition as major analytical instrumentation firms, such as Bruker Corporation and Leica Microsystems, explore quantum upgrades to their optical platforms. The entry of quantum technology companies with vertically integrated supply chains will likely accelerate product development cycles and drive down costs, while also encouraging the adoption of quantum photobiology equipment in both academic and commercial life science laboratories.
Supply Chain and Manufacturing Challenges
The manufacturing of quantum photobiology equipment in 2025 is characterized by a complex supply chain, integrating advanced photonic materials, quantum sensors, and precision electronics. As the industry emerges at the intersection of quantum technology and biological applications, manufacturers are facing significant challenges in sourcing, production, and distribution.
A key challenge is the procurement of quantum-grade photonic components, such as single-photon detectors, entangled photon sources, and ultra-pure optical crystals. Leading suppliers, including Hamamatsu Photonics and Thorlabs, have expanded their offerings to meet demand from quantum life sciences, but limited global capacity and high purity requirements have led to long lead times and increased costs, particularly for custom and small-batch orders.
Semiconductor shortages continue to impact the production of quantum-compatible control electronics and integrated photonic circuits. While some stabilization has occurred since the disruptions of the early 2020s, manufacturers such as Intel and Lumentum are still prioritizing high-volume sectors, causing delays for specialized quantum photobiology equipment producers. This has prompted some companies to seek vertical integration or forge closer partnerships with upstream suppliers to secure critical components.
Precision assembly and calibration are additional bottlenecks. Quantum photobiology devices often require ultra-clean environments and micron-level alignment, necessitating advanced manufacturing infrastructure and highly skilled technicians. Equipment makers like Carl Zeiss AG are investing in automation and AI-based quality control to address these demands, but the shortage of specialized labor remains a persistent issue, especially in regions where quantum manufacturing expertise is nascent.
On a regulatory front, new standards for quantum-enabled medical and biological devices are emerging, adding complexity to compliance and certification processes. Organizations such as ISO are working with industry stakeholders to develop protocols, but the evolving landscape can cause delays in bringing new equipment to market.
Looking ahead, the outlook for supply chain resilience in quantum photobiology equipment manufacturing is cautiously optimistic. Efforts to localize key component production, investments in workforce training, and the adoption of digital supply chain management tools are expected to alleviate some bottlenecks by the late 2020s. However, persistent constraints in rare materials and high-precision photonic components may continue to pose challenges as demand accelerates.
Future Trends & Strategic Opportunities (2025–2030)
The period from 2025 to 2030 is poised to be transformative for the quantum photobiology equipment manufacturing sector, driven by rapid advances in quantum technologies, photonics integration, and the convergence of biology with quantum-enabled instrumentation. Several key trends and strategic opportunities are anticipated to shape the industry landscape in this timeframe.
First, miniaturization and integration of quantum photonic components are expected to accelerate. Manufacturers are investing in scalable fabrication processes for quantum light sources, detectors, and photonic circuits tailored for biological research and clinical diagnostics. Companies such as Hamamatsu Photonics and Thorlabs are expanding their portfolios to include quantum-grade lasers, single-photon counting modules, and ultrafast detectors, which are vital for applications ranging from advanced fluorescence microscopy to optogenetics.
Second, the adoption of quantum sensing platforms in photobiology is set to broaden, driven by demand for ultrasensitive detection of biomolecular events. Equipment manufacturers are collaborating with research institutions to develop quantum-enhanced imaging systems capable of probing cellular processes at unprecedented spatial and temporal resolutions. Carl Zeiss AG and Leica Microsystems are actively exploring integration of quantum optics into next-generation bioimaging platforms, aiming to commercialize instrumentation that leverages entangled photons and quantum coherence for superior signal-to-noise ratios.
Third, the emergence of quantum-ready manufacturing ecosystems is opening new strategic partnerships and supply chain opportunities. Leading photonic foundries and component suppliers are forming alliances with biotechnology firms and academic spin-offs to co-develop application-specific solutions, including chip-scale quantum photonic biosensors and portable diagnostic devices. This collaboration model is expected to lower barriers to market entry and accelerate the translation of quantum photobiology prototypes into deployable equipment.
From a regulatory and standards perspective, industry consortia are working to establish guidelines for the certification and interoperability of quantum-enabled photobiology equipment. Organizations like Optoelectronics Industry Development Association and related international bodies are anticipated to play a pivotal role in harmonizing technical standards, ensuring quality control, and facilitating global market access for advanced equipment.
Overall, the outlook for 2025–2030 suggests robust growth and diversification in the quantum photobiology equipment manufacturing sector, underpinned by technological breakthroughs and cross-disciplinary collaboration. Companies investing in integrated quantum photonic technologies, strategic partnerships, and compliance with emerging standards are well-positioned to capitalize on the expanding opportunities within life sciences, medical diagnostics, and precision biomanufacturing.
Sources & References
- Hamamatsu Photonics
- Thorlabs
- ams OSRAM
- Carl Zeiss AG
- Oxford Instruments
- European Photonics Industry Consortium (EPIC)
- Photonics21
- IBM
- Carl Zeiss AG
- Thorlabs
- European Medicines Agency
- CEN-CENELEC
- International Organization for Standardization
- OSRAM
- Olympus Corporation
- ID Quantique
- Bruker Corporation
- Leica Microsystems
- Lumentum