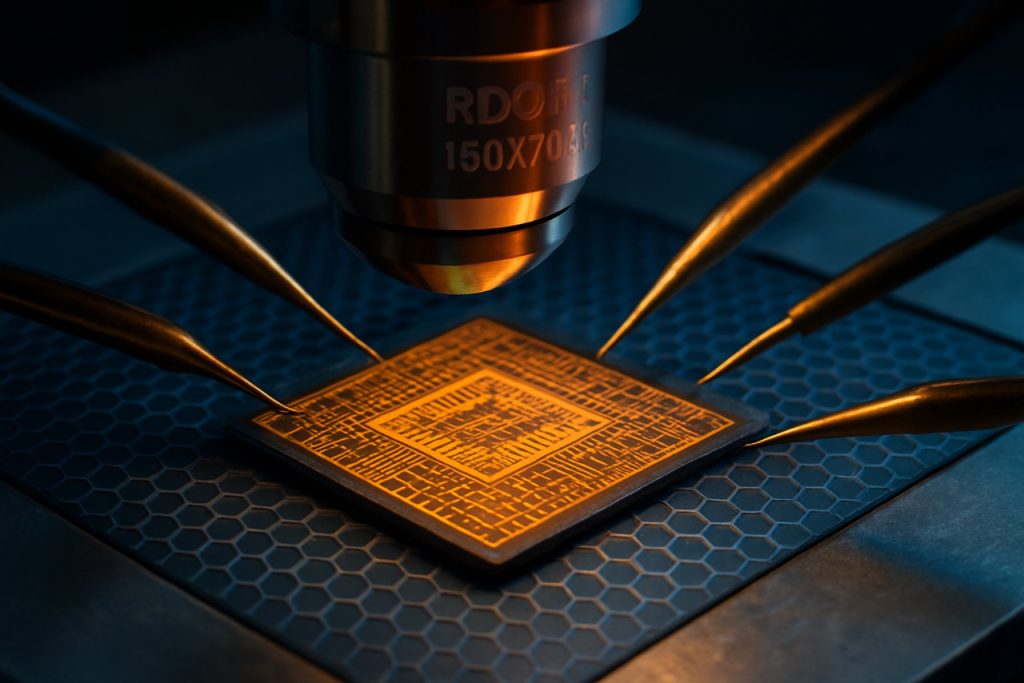
Quantum Junction Nanomaterials Engineering in 2025: Unleashing Breakthroughs in Device Performance and Sustainable Innovation. Explore How Quantum-Driven Materials Are Shaping the Future of Electronics, Energy, and Beyond.
- Executive Summary: Key Trends and Market Drivers in 2025
- Technology Overview: Fundamentals of Quantum Junction Nanomaterials
- Current Market Landscape and Leading Players
- Innovations in Synthesis and Fabrication Techniques
- Applications in Electronics: Transistors, Sensors, and Quantum Computing
- Energy Sector Impact: Photovoltaics, Batteries, and Supercapacitors
- Regulatory Environment and Industry Standards
- Market Forecasts and Growth Projections Through 2030
- Challenges, Risks, and Barriers to Commercialization
- Future Outlook: Emerging Opportunities and Strategic Recommendations
- Sources & References
Executive Summary: Key Trends and Market Drivers in 2025
Quantum junction nanomaterials engineering is poised for significant advancements in 2025, driven by rapid progress in quantum device fabrication, materials innovation, and integration with next-generation electronics. The field focuses on the design and synthesis of nanostructured materials—such as quantum dots, nanowires, and 2D heterostructures—engineered to form quantum junctions with tailored electronic, optical, and spintronic properties. These materials underpin breakthroughs in quantum computing, ultra-efficient photovoltaics, and advanced sensing technologies.
A key trend in 2025 is the scaling of quantum dot and nanowire production for device integration. Companies like Nanosys and Nanoco Group are expanding their manufacturing capabilities to supply quantum dot materials for both display and emerging quantum information applications. These firms are investing in environmentally friendly, heavy-metal-free quantum dots, addressing regulatory and sustainability concerns while meeting the performance demands of quantum junction devices.
Another major driver is the convergence of nanomaterials engineering with semiconductor manufacturing. Industry leaders such as Intel and IBM are actively developing quantum junction architectures using silicon, germanium, and compound semiconductor nanostructures. Their research and pilot lines are targeting scalable quantum processors and hybrid classical-quantum chips, with a focus on reproducibility and integration with existing CMOS infrastructure.
In the energy sector, quantum junction nanomaterials are enabling new generations of high-efficiency solar cells and photodetectors. Companies like First Solar are exploring quantum dot and perovskite nanomaterial layers to surpass traditional efficiency limits and improve low-light performance. These innovations are expected to reach pilot commercialization within the next few years, with potential to disrupt both utility-scale and distributed solar markets.
The outlook for 2025 and beyond is shaped by increased public and private investment in quantum materials R&D, as well as the formation of global consortia to standardize materials and device interfaces. Organizations such as Semiconductor Industry Association are facilitating collaboration between material suppliers, device manufacturers, and research institutions to accelerate commercialization and address supply chain challenges.
In summary, the quantum junction nanomaterials engineering sector in 2025 is characterized by rapid scaling, cross-industry collaboration, and a focus on sustainable, high-performance materials. These trends are expected to drive the adoption of quantum-enabled devices across computing, energy, and sensing markets, setting the stage for transformative technological advances in the coming years.
Technology Overview: Fundamentals of Quantum Junction Nanomaterials
Quantum junction nanomaterials engineering is a rapidly advancing field at the intersection of quantum physics, materials science, and nanoscale device fabrication. The core concept involves the deliberate design and synthesis of nanostructures—such as quantum dots, nanowires, and heterostructures—where quantum mechanical effects dominate charge, spin, and energy transport across engineered junctions. These quantum junctions, often formed at the interface of dissimilar nanomaterials, enable novel functionalities that are unattainable in bulk or classical systems.
In 2025, the field is characterized by a strong focus on controlling interfacial properties at the atomic and molecular level. Researchers are leveraging advanced epitaxial growth techniques, atomic layer deposition, and precision lithography to fabricate quantum junctions with unprecedented uniformity and reproducibility. For example, the integration of III-V semiconductor quantum dots with two-dimensional materials like graphene and transition metal dichalcogenides (TMDs) is enabling new classes of optoelectronic and quantum information devices. Companies such as Samsung Electronics and Taiwan Semiconductor Manufacturing Company are actively developing quantum dot and nanowire-based device platforms, with a focus on scalable manufacturing and integration with existing semiconductor processes.
A key technological milestone in 2025 is the demonstration of high-efficiency quantum junction solar cells and photodetectors, where engineered nanomaterial interfaces facilitate enhanced charge separation and reduced recombination losses. First Solar and National Renewable Energy Laboratory are among the organizations pushing the boundaries of quantum junction photovoltaics, reporting device architectures that surpass traditional efficiency limits by exploiting quantum confinement and hot-carrier extraction mechanisms.
In parallel, quantum junction nanomaterials are being engineered for next-generation quantum computing and sensing applications. Superconducting and semiconducting nanowire junctions, for instance, are central to the development of topological qubits and ultra-sensitive magnetometers. IBM and Intel Corporation are investing in scalable quantum device fabrication, with a particular emphasis on hybrid nanomaterial junctions that combine coherence, tunability, and integration potential.
Looking ahead, the outlook for quantum junction nanomaterials engineering is robust. The next few years are expected to see further advances in atomic-scale interface control, defect engineering, and large-area synthesis. These developments will be critical for the commercialization of quantum-enabled devices in computing, energy, and sensing. Industry collaborations and consortia, such as those led by Semiconductor Industry Association, are likely to accelerate the translation of laboratory breakthroughs into manufacturable technologies, setting the stage for a new era of quantum-enabled nanodevices.
Current Market Landscape and Leading Players
Quantum junction nanomaterials engineering is rapidly evolving, driven by advances in quantum dot (QD) synthesis, heterostructure design, and scalable manufacturing. As of 2025, the market landscape is shaped by a convergence of established semiconductor giants, specialized nanomaterials firms, and emerging startups, all vying to commercialize next-generation optoelectronic, photovoltaic, and quantum information technologies.
A key trend is the integration of quantum junction nanomaterials into high-efficiency solar cells and displays. Companies such as Samsung Electronics and LG Electronics have continued to invest in quantum dot-enhanced displays, leveraging engineered nanomaterial junctions to achieve superior color purity and energy efficiency. In photovoltaics, First Solar is exploring quantum junction architectures to push the boundaries of thin-film solar cell efficiency, while Nanoco Group specializes in cadmium-free quantum dots for both display and solar applications.
In the quantum computing and sensing domain, IBM and Intel are actively developing quantum junction nanomaterials as part of their quantum processor roadmaps, focusing on materials that enable stable qubit operation and scalable device integration. QD Laser in Japan is commercializing quantum dot-based laser diodes, which rely on precise nanomaterial junction engineering for telecom and medical applications.
Startups and research spinouts are also shaping the competitive landscape. Nanosys is a leading supplier of quantum dot materials for displays, collaborating with major OEMs to integrate advanced nanomaterial junctions into consumer electronics. Quantum Solutions and Avantama are notable for their work on solution-processable quantum junction inks, targeting printable electronics and flexible devices.
Looking ahead, the market is expected to see increased collaboration between material suppliers and device manufacturers, with a focus on scaling up environmentally friendly, heavy-metal-free quantum junction nanomaterials. Regulatory and supply chain considerations, especially regarding rare earth and toxic elements, are influencing R&D priorities. The next few years will likely witness the commercialization of quantum junction nanomaterials in new sectors, including quantum communication and advanced biosensing, as companies like Oxford Instruments expand their nanofabrication tool offerings to support these emerging applications.
Innovations in Synthesis and Fabrication Techniques
Quantum junction nanomaterials engineering is experiencing rapid advancements in synthesis and fabrication techniques, driven by the demand for high-performance optoelectronic devices, quantum computing components, and next-generation sensors. In 2025, the focus is on scalable, reproducible, and cost-effective methods that enable precise control over nanomaterial interfaces and quantum properties.
One of the most significant innovations is the adoption of atomic layer deposition (ALD) and molecular beam epitaxy (MBE) for constructing quantum junctions with atomic precision. These techniques allow for the layer-by-layer assembly of heterostructures, enabling the engineering of band alignments and interface properties critical for quantum tunneling and carrier transport. Companies such as Oxford Instruments and Veeco Instruments are at the forefront, supplying advanced ALD and MBE systems tailored for research and pilot-scale production of quantum nanomaterials.
Colloidal synthesis methods have also matured, particularly for quantum dots and nanorods, allowing for tunable size, shape, and surface chemistry. This is essential for creating quantum junctions with tailored electronic and optical properties. Nanosys and Nanoco Technologies are notable for their scalable production of quantum dots, which are increasingly integrated into quantum junction devices for displays, photovoltaics, and photodetectors.
A key trend in 2025 is the integration of two-dimensional (2D) materials, such as transition metal dichalcogenides (TMDs) and graphene, into quantum junction architectures. Techniques like chemical vapor deposition (CVD) and van der Waals stacking are being refined to produce high-quality, large-area 2D heterostructures. Graphenea and 2D Semiconductors are recognized suppliers of 2D materials, supporting both academic and industrial R&D in this area.
Looking ahead, the outlook for quantum junction nanomaterials engineering is promising. The convergence of precision synthesis, in-situ characterization, and machine learning-driven process optimization is expected to accelerate the discovery and commercialization of novel quantum devices. Industry collaborations and pilot manufacturing lines, such as those supported by imec, are anticipated to bridge the gap between laboratory-scale innovation and industrial-scale production, paving the way for widespread adoption of quantum-enabled technologies in the coming years.
Applications in Electronics: Transistors, Sensors, and Quantum Computing
Quantum junction nanomaterials engineering is rapidly transforming the landscape of electronic device applications, particularly in transistors, sensors, and quantum computing. As of 2025, the integration of quantum junctions—interfaces where quantum effects dominate charge transport—into nanomaterials is enabling unprecedented device miniaturization, sensitivity, and computational power.
In the field of transistors, quantum junction nanomaterials are at the forefront of next-generation device scaling. Companies such as Intel Corporation and Samsung Electronics are actively developing gate-all-around (GAA) transistor architectures that leverage nanowire and nanosheet channels, where quantum confinement and tunneling effects at junctions are critical for performance at sub-3nm nodes. These advances are expected to enter high-volume manufacturing by 2025–2026, with early prototypes already demonstrating improved drive current and reduced leakage compared to traditional FinFETs. The use of engineered heterojunctions and atomically precise interfaces is also being explored to further suppress short-channel effects and enable even smaller device geometries.
Sensor technology is another area witnessing significant impact from quantum junction nanomaterials. The unique electronic and optical properties at quantum junctions—such as enhanced carrier mobility and tunable band alignment—are being harnessed to create highly sensitive photodetectors, biosensors, and gas sensors. imec, a leading nanoelectronics research institute, is collaborating with industry partners to develop 2D material-based sensor arrays that exploit quantum tunneling at junctions for single-molecule detection and ultra-low power operation. These sensors are expected to find applications in medical diagnostics, environmental monitoring, and wearable electronics within the next few years.
Quantum computing represents perhaps the most transformative application of quantum junction nanomaterials. Companies such as IBM and Intel Corporation are advancing the fabrication of quantum dots, Josephson junctions, and topological qubits using precisely engineered nanomaterials. The control of quantum coherence and entanglement at these junctions is essential for scalable quantum processors. In 2025, both companies are targeting the demonstration of quantum processors with over 1,000 physical qubits, leveraging advances in nanomaterial engineering to improve qubit fidelity and connectivity. The outlook for the next few years includes the integration of quantum junction nanomaterials into hybrid classical-quantum systems, paving the way for practical quantum advantage in select computational tasks.
Overall, the convergence of quantum junction engineering and nanomaterials is poised to redefine the boundaries of electronics, with major industry players and research institutes accelerating the transition from laboratory breakthroughs to commercial technologies across transistors, sensors, and quantum computing platforms.
Energy Sector Impact: Photovoltaics, Batteries, and Supercapacitors
Quantum junction nanomaterials engineering is rapidly transforming the energy sector, particularly in photovoltaics, batteries, and supercapacitors. As of 2025, the integration of quantum dots, nanowires, and heterostructured materials at junctions is enabling significant advances in device efficiency, stability, and scalability.
In photovoltaics, quantum junctions—interfaces engineered at the nanoscale between different semiconductor materials—are driving the next generation of solar cells. Quantum dot-sensitized solar cells (QDSSCs) and perovskite–quantum dot tandem architectures are achieving power conversion efficiencies (PCE) exceeding 20%, with some laboratory prototypes approaching 25%. Companies such as First Solar and Nanoco Group are actively developing quantum dot and nanomaterial-based photovoltaic modules, focusing on scalable manufacturing and environmental safety. The use of engineered quantum junctions allows for tunable bandgaps, improved charge separation, and reduced recombination losses, which are critical for high-efficiency solar panels.
In the battery sector, quantum junction nanomaterials are being employed to enhance both lithium-ion and emerging solid-state battery technologies. Nanoscale engineering at electrode–electrolyte interfaces improves ion transport and mitigates dendrite formation, a key challenge for high-energy-density batteries. Samsung Electronics and Toshiba Corporation are among the companies exploring nanostructured anodes and cathodes, leveraging quantum effects to increase cycle life and charging speed. For example, quantum dot coatings on silicon anodes have demonstrated improved structural stability and higher capacity retention over hundreds of cycles.
Supercapacitors are also benefiting from quantum junction nanomaterials, with research and pilot-scale production focusing on hybrid devices that combine the high power density of capacitors with the energy density of batteries. Companies like Maxwell Technologies (a subsidiary of Tesla) are investigating graphene–quantum dot composites and heterostructured electrodes to achieve rapid charge/discharge rates and long operational lifetimes. These materials enable the design of supercapacitors with energy densities exceeding 30 Wh/kg, narrowing the gap with traditional batteries while maintaining superior power performance.
Looking ahead, the next few years are expected to see further commercialization of quantum junction nanomaterial technologies, driven by advances in scalable synthesis, device integration, and lifecycle management. Industry collaborations and government initiatives are accelerating the transition from laboratory-scale breakthroughs to market-ready products, with a strong emphasis on sustainability and supply chain resilience. As quantum junction engineering matures, it is poised to play a pivotal role in the global shift toward renewable energy and electrification.
Regulatory Environment and Industry Standards
The regulatory environment and industry standards for quantum junction nanomaterials engineering are rapidly evolving as the field matures and commercial applications expand. In 2025, the focus is on harmonizing safety, quality, and performance standards to support the integration of quantum nanomaterials into sectors such as electronics, energy, and healthcare.
Key regulatory bodies, including the International Organization for Standardization (ISO) and the International Electrotechnical Commission (IEC), are actively developing and updating standards specific to nanomaterials and quantum-enabled devices. ISO’s Technical Committee 229 (Nanotechnologies) is working on guidelines for characterization, risk assessment, and lifecycle management of nanomaterials, which are directly relevant to quantum junction materials. The IEC, through its Technical Committee 113, is addressing standardization for nanotechnology in electrical and electronic products, including quantum dot and quantum well structures.
In the United States, the National Institute of Standards and Technology (NIST) is collaborating with industry leaders to establish measurement protocols and reference materials for quantum nanomaterials, aiming to ensure reproducibility and interoperability across the supply chain. The U.S. Environmental Protection Agency (EPA) continues to monitor the environmental and health impacts of engineered nanomaterials, with a particular emphasis on lifecycle analysis and end-of-life disposal of quantum-enabled products.
On the industry side, major manufacturers such as Samsung Electronics and Taiwan Semiconductor Manufacturing Company (TSMC) are participating in consortia to define best practices for the fabrication and integration of quantum junction nanomaterials in next-generation semiconductors. These companies are also engaging with the Semiconductor Industry Association (SIA) to advocate for globally aligned standards that facilitate international trade and innovation.
Looking ahead, the next few years are expected to see the introduction of more comprehensive certification schemes and labeling requirements for quantum nanomaterial-based products, particularly in consumer electronics and medical devices. The European Union is anticipated to expand its regulatory framework under REACH (Registration, Evaluation, Authorisation and Restriction of Chemicals) to explicitly address quantum nanomaterials, following ongoing consultations with stakeholders and scientific committees.
Overall, the regulatory landscape in 2025 is characterized by increased coordination between international standards bodies, national regulators, and industry leaders. This collaborative approach is essential to address the unique challenges posed by quantum junction nanomaterials, ensuring both innovation and public safety as the technology moves toward broader commercialization.
Market Forecasts and Growth Projections Through 2030
The market for quantum junction nanomaterials engineering is poised for significant expansion through 2030, driven by rapid advancements in quantum dot, nanowire, and heterostructure technologies. As of 2025, the sector is witnessing increased investment from both established semiconductor manufacturers and emerging nanomaterials specialists, with a focus on applications in next-generation electronics, optoelectronics, and quantum computing.
Key industry players such as Samsung Electronics and Intel Corporation are actively developing quantum junction-based devices, leveraging their expertise in nanoscale fabrication and integration. Samsung Electronics has announced ongoing research into quantum dot-based transistors and memory elements, aiming to enhance device performance and energy efficiency. Similarly, Intel Corporation is investing in quantum junction architectures for scalable quantum processors, with pilot production lines expected to mature by 2027.
In the materials supply chain, companies like BASF and 3M are scaling up the synthesis and functionalization of nanomaterials tailored for quantum junction applications. BASF is expanding its nanomaterials portfolio to include high-purity quantum dots and nanowires, targeting both the electronics and photonics sectors. 3M is focusing on advanced encapsulation and integration solutions to improve the stability and manufacturability of quantum junction devices.
The outlook for the next five years indicates a compound annual growth rate (CAGR) in the high teens for quantum junction nanomaterials, with the strongest demand projected in quantum computing, high-efficiency photovoltaics, and advanced display technologies. The adoption of quantum junctions in photodetectors and light-emitting devices is expected to accelerate, as companies such as OSRAM and TDK Corporation integrate these materials into commercial products.
By 2030, the market is anticipated to be shaped by breakthroughs in scalable manufacturing, improved material uniformity, and integration with existing semiconductor processes. Strategic partnerships between material suppliers, device manufacturers, and research institutions are likely to drive innovation and commercialization. As regulatory frameworks and industry standards evolve, the sector is expected to transition from pilot-scale demonstrations to widespread adoption in consumer and industrial applications.
Challenges, Risks, and Barriers to Commercialization
Quantum junction nanomaterials engineering, a field at the intersection of quantum physics and advanced materials science, is rapidly progressing toward commercial applications in electronics, photonics, and energy devices. However, as of 2025, several significant challenges, risks, and barriers continue to impede widespread commercialization.
A primary technical challenge lies in the reproducible synthesis and large-scale manufacturing of quantum junction nanomaterials with precise control over their size, composition, and interface quality. Quantum effects are highly sensitive to atomic-scale defects and interface disorder, which can degrade device performance. For example, companies such as Samsung Electronics and Taiwan Semiconductor Manufacturing Company are investing in advanced fabrication techniques, but consistently achieving defect-free quantum junctions at wafer scale remains a formidable hurdle.
Material stability and environmental sensitivity also pose risks. Many quantum nanomaterials, such as perovskite quantum dots and 2D transition metal dichalcogenides, are prone to degradation when exposed to air, moisture, or elevated temperatures. This limits their operational lifetimes and reliability in commercial products. Merck KGaA, a supplier of specialty chemicals and nanomaterials, is actively developing encapsulation and passivation strategies, but robust, scalable solutions are still under development.
Another barrier is the integration of quantum junction nanomaterials with existing semiconductor manufacturing infrastructure. The compatibility of new materials with established CMOS processes is critical for cost-effective adoption. Leading foundries like Intel Corporation and GlobalFoundries are exploring hybrid integration schemes, but process standardization and yield optimization are ongoing concerns.
Intellectual property (IP) complexity and regulatory uncertainty further complicate commercialization. The rapid pace of innovation has led to a fragmented IP landscape, with overlapping patents and unclear freedom-to-operate for startups and established players alike. Additionally, regulatory frameworks for the environmental and health impacts of nanomaterials are still evolving, with organizations such as U.S. Environmental Protection Agency and Organisation for Economic Co-operation and Development working to establish guidelines for safe handling and disposal.
Looking ahead, overcoming these challenges will require coordinated efforts in materials research, process engineering, and regulatory policy. Industry consortia and public-private partnerships are expected to play a key role in addressing technical and standardization barriers, while ongoing advances in characterization and in-situ monitoring may help accelerate the transition from laboratory-scale demonstrations to reliable, manufacturable quantum junction nanomaterials for commercial devices.
Future Outlook: Emerging Opportunities and Strategic Recommendations
Quantum junction nanomaterials engineering is poised for significant advancements in 2025 and the coming years, driven by rapid progress in quantum device fabrication, materials synthesis, and integration with classical electronics. The convergence of quantum materials—such as 2D transition metal dichalcogenides, topological insulators, and engineered heterostructures—at the nanoscale is enabling the creation of quantum junctions with unprecedented control over electron transport, spin, and coherence properties.
Key industry players are accelerating the translation of laboratory breakthroughs into scalable technologies. IBM continues to invest in quantum materials research, focusing on the development of hybrid quantum-classical architectures and the integration of quantum junctions into superconducting and semiconducting qubit platforms. Intel is advancing silicon-based quantum dot arrays, leveraging its expertise in nanoscale fabrication to engineer precise quantum junctions for scalable quantum processors. Meanwhile, Toshiba is exploring quantum dot and nanowire junctions for quantum communication and cryptography applications, aiming to commercialize secure quantum networks.
In 2025, the field is expected to see the first commercial deployments of quantum-enhanced sensors and photonic devices utilizing engineered nanomaterial junctions. For example, Hitachi High-Tech is developing quantum tunneling-based sensors for ultra-sensitive detection in medical diagnostics and environmental monitoring. These devices exploit the unique properties of quantum junctions to achieve sensitivity and selectivity beyond classical limits.
Strategically, companies are forming cross-disciplinary partnerships to address challenges in reproducibility, scalability, and integration. Collaborative initiatives between industry and academic institutions are focusing on standardizing nanomaterial synthesis and junction fabrication processes, as well as developing robust characterization techniques. The emergence of open innovation platforms and consortia, such as those led by IBM and Intel, is expected to accelerate the pace of innovation and reduce time-to-market for new quantum junction-based products.
Looking ahead, the next few years will likely see increased investment in pilot manufacturing lines for quantum nanomaterial devices, with a focus on quantum computing, secure communications, and advanced sensing. Companies that prioritize intellectual property development, supply chain resilience, and workforce training in quantum nanotechnology will be best positioned to capitalize on emerging opportunities. Strategic recommendations include fostering ecosystem partnerships, investing in scalable fabrication infrastructure, and engaging with standardization bodies to shape the regulatory landscape for quantum nanomaterials engineering.
Sources & References
- IBM
- First Solar
- Semiconductor Industry Association
- National Renewable Energy Laboratory
- LG Electronics
- QD Laser
- Quantum Solutions
- Avantama
- Oxford Instruments
- Oxford Instruments
- Veeco Instruments
- 2D Semiconductors
- imec
- Toshiba Corporation
- Maxwell Technologies
- International Organization for Standardization
- National Institute of Standards and Technology
- BASF
- OSRAM
- IBM
- Hitachi High-Tech