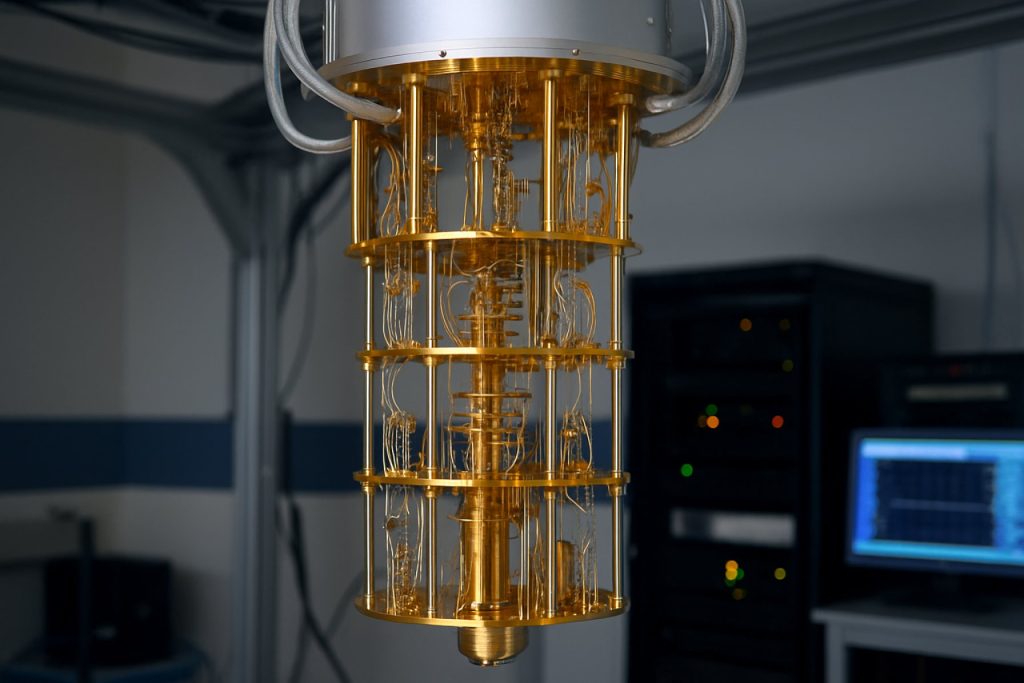
Unlocking Quantum Potential: How Cryogenic Engineering Will Shape Quantum Computing in 2025 and Beyond. Explore the Technologies, Market Growth, and Strategic Shifts Driving the Next Era of Ultra-Cold Quantum Systems.
- Executive Summary: Cryogenics as the Backbone of Quantum Computing
- Market Size and Growth Forecast (2025–2030): CAGR and Revenue Projections
- Key Cryogenic Technologies Powering Quantum Processors
- Major Players and Strategic Partnerships (e.g., Bluefors, Oxford Instruments, IBM, Google)
- Supply Chain and Manufacturing Trends in Cryogenic Systems
- Technical Challenges: Thermal Management, Scalability, and Reliability
- Emerging Applications: Quantum Data Centers, Communications, and Sensing
- Regulatory, Safety, and Standardization Initiatives (IEEE, ASME)
- Investment Landscape: Funding, M&A, and Startup Ecosystem
- Future Outlook: Disruptive Innovations and Long-Term Market Impact
- Sources & References
Executive Summary: Cryogenics as the Backbone of Quantum Computing
Cryogenic engineering has rapidly emerged as a foundational pillar in the advancement of quantum computing, particularly as the industry moves into 2025 and beyond. Quantum processors—especially those based on superconducting qubits and spin qubits—require ultra-low temperatures, often below 20 millikelvin, to maintain quantum coherence and minimize noise. This necessity has driven significant innovation and investment in cryogenic technologies, positioning them as the backbone of scalable quantum computing infrastructure.
The current landscape is shaped by a handful of specialized manufacturers and technology leaders. Bluefors, headquartered in Finland, is widely recognized as a global leader in the production of dilution refrigerators, which are essential for cooling quantum processors. Their systems are deployed in major quantum research labs and commercial quantum computing facilities worldwide. Similarly, Oxford Instruments in the UK has a long-standing reputation for delivering advanced cryogenic and superconducting solutions, supporting both academic and industrial quantum initiatives.
In the United States, Quantum Machines and JanisULT (a division of Lake Shore Cryotronics) are notable for their integrated cryogenic platforms and control systems, which are increasingly being adopted by quantum hardware developers. These companies are not only improving the reliability and scalability of cryogenic systems but are also working to reduce operational complexity and energy consumption—key factors as quantum computers transition from laboratory prototypes to commercial products.
The demand for robust cryogenic infrastructure is further underscored by the activities of quantum computing giants such as IBM and Rigetti Computing, both of which have made public commitments to scaling up their quantum processors. IBM, for example, has announced plans to develop quantum systems with thousands of qubits by the late 2020s, a goal that will require unprecedented advances in cryogenic engineering to ensure stable, long-term operation of large-scale quantum devices.
Looking ahead, the next few years are expected to see continued collaboration between cryogenic specialists and quantum hardware developers. Innovations such as automated cryostat management, improved thermal anchoring, and integration with classical control electronics are anticipated to further streamline quantum system deployment. As quantum computing edges closer to practical utility, the role of cryogenic engineering will only grow in importance, cementing its status as the technological backbone of the field.
Market Size and Growth Forecast (2025–2030): CAGR and Revenue Projections
The market for cryogenic engineering in quantum computing is poised for significant expansion between 2025 and 2030, driven by the rapid advancement and commercialization of quantum technologies. Cryogenic systems are essential for maintaining the ultra-low temperatures required by leading quantum computing modalities, such as superconducting qubits and spin qubits, which typically operate at millikelvin ranges. As quantum computing transitions from laboratory research to early-stage commercial deployment, demand for high-reliability, scalable cryogenic infrastructure is accelerating.
Key industry players, including Bluefors, Oxford Instruments, and Linde, are investing heavily in next-generation dilution refrigerators, cryostats, and cryogenic support systems tailored for quantum computing applications. Bluefors, for example, is recognized as a global leader in cryogenic platforms for quantum research and has reported a surge in orders from both academic and commercial quantum computing initiatives. Oxford Instruments continues to expand its product portfolio, focusing on modular and scalable cryogenic solutions to meet the evolving needs of quantum hardware developers.
While precise market sizing figures for cryogenic engineering specific to quantum computing are not universally published, industry consensus and company disclosures suggest a robust compound annual growth rate (CAGR) in the range of 20–30% through 2030. This growth is underpinned by increasing investments from quantum hardware companies, national quantum initiatives, and cloud service providers integrating quantum processors into their infrastructure. For instance, IBM and Google have both announced plans to scale up their quantum computing capabilities, which directly translates to increased procurement of advanced cryogenic systems.
Revenue projections for the global cryogenic engineering market in quantum computing are expected to reach several hundred million USD by 2030, with some industry estimates placing the figure above $500 million, depending on the pace of quantum hardware commercialization and the adoption of cryogenic solutions in emerging quantum data centers. The outlook for 2025–2030 is further strengthened by ongoing collaborations between cryogenic equipment manufacturers and quantum technology firms, as well as government-backed quantum programs in the US, Europe, and Asia-Pacific.
In summary, the period from 2025 to 2030 is anticipated to witness rapid growth in the cryogenic engineering market for quantum computing, characterized by double-digit CAGR, expanding revenue pools, and increasing strategic partnerships among leading cryogenic and quantum technology companies.
Key Cryogenic Technologies Powering Quantum Processors
Cryogenic engineering is a foundational pillar for quantum computing, as most quantum processors—especially those based on superconducting qubits and spin qubits—require operation at temperatures close to absolute zero. In 2025, the field is witnessing rapid advancements in both the performance and scalability of cryogenic systems, driven by the demands of quantum hardware developers and the emergence of commercial quantum computing platforms.
The dominant technology for cooling quantum processors is the dilution refrigerator, which can achieve temperatures below 10 millikelvin. Leading manufacturers such as Bluefors Oy and Oxford Instruments have become central suppliers to quantum computing companies and research institutions worldwide. These companies are innovating in refrigerator design to support larger payloads, higher cooling power, and improved integration with quantum control electronics. For example, Bluefors Oy has introduced modular cryogenic platforms that facilitate the scaling of quantum processors to hundreds or even thousands of qubits, while maintaining the ultra-low temperatures required for quantum coherence.
Another key trend in 2025 is the integration of cryogenic-compatible electronics. As quantum processors scale, the need to minimize thermal load from wiring and control hardware becomes critical. Companies such as Intel Corporation are developing cryogenic CMOS (complementary metal-oxide-semiconductor) control chips that can operate at temperatures below 4 kelvin, reducing the number of wires entering the cryostat and enabling more efficient scaling. This approach is expected to be a major enabler for practical, large-scale quantum computers in the coming years.
Additionally, the industry is exploring alternative cooling technologies to address the limitations of traditional dilution refrigerators. Pulse-tube cryocoolers, offered by companies like Cryomech Inc., are being adopted for their reliability and reduced maintenance requirements, particularly in commercial and cloud-based quantum computing services. These systems are often used in conjunction with dilution refrigerators to pre-cool stages and improve overall system efficiency.
Looking ahead, the outlook for cryogenic engineering in quantum computing is marked by a push toward greater automation, remote monitoring, and modularity. Companies are investing in smart cryogenic systems with integrated diagnostics and remote control capabilities, aiming to support 24/7 operation in data center environments. As quantum processors continue to grow in complexity, the demand for robust, scalable, and user-friendly cryogenic infrastructure will remain a key driver of innovation in the sector.
Major Players and Strategic Partnerships (e.g., Bluefors, Oxford Instruments, IBM, Google)
The landscape of cryogenic engineering for quantum computing in 2025 is defined by a handful of major players and a growing web of strategic partnerships. These collaborations are crucial, as the technical demands of quantum processors—especially those based on superconducting qubits—require ultra-low temperatures, often below 20 millikelvin, achievable only with advanced dilution refrigerators and cryogenic infrastructure.
Among the most prominent companies is Bluefors, a Finnish manufacturer specializing in cryogenic measurement systems. Bluefors has become a global leader in dilution refrigerators, supplying systems to both academic and industrial quantum computing labs. Their modular platforms are designed for scalability, a key requirement as quantum processors increase in qubit count. In recent years, Bluefors has announced partnerships with leading quantum computing companies and research institutions, aiming to co-develop next-generation cryogenic solutions tailored for large-scale quantum computers.
Another major player is Oxford Instruments, a UK-based company with a long history in cryogenics and scientific instrumentation. Oxford Instruments provides a range of cryofree dilution refrigerators and has been actively collaborating with quantum hardware developers to optimize system integration and performance. Their platforms are widely used in both commercial and government quantum initiatives, and the company continues to invest in automation and remote monitoring capabilities to support the operational needs of quantum data centers.
On the end-user side, technology giants such as IBM and Google are not only developing quantum processors but also investing heavily in cryogenic engineering. IBM’s “Quantum System One” and “Quantum System Two” platforms integrate custom cryogenic infrastructure, much of it developed in partnership with leading suppliers. IBM has also announced collaborations with Bluefors and Oxford Instruments to push the boundaries of cryogenic performance and reliability. Google, meanwhile, has built custom cryogenic labs to support its Sycamore and future quantum processors, and is known to work closely with both Bluefors and Oxford Instruments for its hardware needs.
Strategic partnerships are expanding beyond traditional supplier-customer relationships. For example, Bluefors and Oxford Instruments have both entered into joint development agreements with quantum hardware startups and national laboratories, aiming to address challenges such as cryogenic cabling, thermal management, and system automation. These alliances are expected to accelerate the deployment of larger, more stable quantum systems over the next few years.
Looking ahead, the interplay between these major players and their partners will be pivotal in overcoming the engineering bottlenecks of scaling quantum computers. As quantum processors approach the 1,000-qubit mark and beyond, the demand for robust, scalable, and automated cryogenic solutions will only intensify, driving further innovation and collaboration across the sector.
Supply Chain and Manufacturing Trends in Cryogenic Systems
The supply chain and manufacturing landscape for cryogenic systems in quantum computing is undergoing significant transformation as the sector matures in 2025. The demand for ultra-low temperature environments—often below 10 millikelvin—remains a critical enabler for superconducting and spin-based quantum processors. This has led to a surge in both the scale and sophistication of cryogenic engineering, with a focus on reliability, modularity, and integration with quantum hardware.
Key industry players such as Bluefors and Oxford Instruments continue to dominate the market for dilution refrigerators, which are the backbone of most quantum computing platforms. Both companies have expanded their manufacturing capacities and introduced new product lines tailored for quantum computing, emphasizing higher cooling power, reduced vibration, and improved system automation. In 2024 and 2025, Bluefors announced partnerships with leading quantum hardware developers to co-design cryogenic platforms, aiming to streamline integration and reduce time-to-deployment.
Supply chain resilience has become a focal point, especially in the wake of global semiconductor and helium shortages. Manufacturers are increasingly localizing component sourcing and investing in vertical integration. For example, Oxford Instruments has reported efforts to secure critical supply lines for rare materials and custom components, while also developing in-house capabilities for key subsystems such as pulse tube coolers and cryogenic wiring.
Emerging entrants like Linde and Cryomech are leveraging their expertise in industrial gases and cryocoolers, respectively, to offer scalable solutions for quantum labs and data centers. Linde is focusing on helium recovery and liquefaction systems, addressing both cost and sustainability concerns, while Cryomech is advancing pulse tube cryocooler technology to support continuous operation and minimize maintenance.
Looking ahead, the next few years are expected to see further standardization of cryogenic interfaces and increased adoption of modular, plug-and-play systems. Industry consortia and collaborations with quantum hardware companies are driving the development of open standards for cryogenic interconnects and monitoring, which will facilitate interoperability and accelerate deployment. Additionally, automation and remote diagnostics are being integrated into new systems, enabling predictive maintenance and reducing the need for specialized onsite personnel.
Overall, the cryogenic supply chain for quantum computing is evolving rapidly, with established manufacturers scaling up, new entrants innovating, and the entire ecosystem moving toward greater resilience, efficiency, and integration.
Technical Challenges: Thermal Management, Scalability, and Reliability
Cryogenic engineering is a cornerstone of quantum computing, enabling the ultra-low temperatures required for the operation of superconducting qubits and other quantum devices. As the quantum computing sector advances into 2025, the technical challenges of thermal management, scalability, and reliability are increasingly prominent, shaping both research priorities and commercial strategies.
Thermal Management: Quantum processors, particularly those based on superconducting circuits, must operate at temperatures near absolute zero—typically below 20 millikelvin. Achieving and maintaining these temperatures requires sophisticated dilution refrigerators. Leading manufacturers such as Bluefors Oy and Oxford Instruments plc have become central to the industry, supplying cryogenic systems to major quantum computing companies. In 2025, the focus is on improving cooling power, reducing thermal noise, and integrating more efficient heat exchangers to support larger quantum processors. The challenge is compounded by the need to manage heat loads from control wiring and amplifiers, which increase as systems scale up.
Scalability: As quantum computers move from tens to hundreds or thousands of qubits, the cryogenic infrastructure must scale accordingly. This involves not only larger refrigerators but also innovations in cryogenic cabling, filtering, and signal routing. Companies like Lake Shore Cryotronics, Inc. are developing advanced cryogenic measurement and control solutions to address these needs. The integration of cryogenic electronics—such as low-temperature amplifiers and multiplexers—is a key area of research, aiming to reduce the number of physical connections between room temperature and the quantum processor, thus minimizing heat influx and complexity.
Reliability: Long-term, stable operation of cryogenic systems is essential for both research and commercial quantum computing. Unplanned thermal cycling or system downtime can disrupt experiments and damage sensitive components. In response, manufacturers are enhancing system automation, remote monitoring, and predictive maintenance capabilities. For example, Bluefors Oy and Oxford Instruments plc are investing in software and hardware solutions to improve uptime and reduce manual intervention. Additionally, the reliability of cryogenic components—such as vacuum seals, pumps, and wiring—remains a focus, with ongoing efforts to extend service intervals and reduce failure rates.
Looking ahead, the next few years will see continued collaboration between quantum hardware developers and cryogenic engineering firms. The push for larger, more reliable quantum computers will drive innovation in cryogenic system design, with an emphasis on modularity, automation, and integration of cryogenic electronics. As the industry matures, the ability to deliver robust, scalable, and efficient cryogenic infrastructure will be a key differentiator for both quantum computing providers and their cryogenic partners.
Emerging Applications: Quantum Data Centers, Communications, and Sensing
Cryogenic engineering is rapidly becoming a cornerstone for the next generation of quantum technologies, particularly as quantum computing transitions from laboratory prototypes to scalable, commercially viable systems. In 2025 and the coming years, the demand for advanced cryogenic solutions is intensifying, driven by the emergence of quantum data centers, quantum communication networks, and quantum sensing applications.
Quantum computers, especially those based on superconducting qubits and spin qubits, require stable operation at temperatures close to absolute zero—often below 20 millikelvin. This necessitates sophisticated dilution refrigerators and cryogenic infrastructure. Leading manufacturers such as Bluefors and Oxford Instruments are at the forefront, supplying modular, scalable cryogenic platforms tailored for multi-qubit systems. In 2024, Bluefors announced new high-capacity cryostats designed for quantum data centers, supporting hundreds of qubits and integrating with automated control systems to minimize downtime and maintenance.
The rise of quantum data centers—dedicated facilities for hosting quantum processors—has placed new demands on cryogenic engineering. These centers require not only reliable ultra-low temperature environments but also efficient thermal management, vibration isolation, and electromagnetic shielding. Companies like IBM and Leiden Cryogenics are investing in next-generation cryogenic infrastructure to support their quantum cloud services and research platforms. IBM’s Quantum System Two, for example, features a modular cryogenic architecture designed for continuous operation and rapid scaling, with the goal of supporting thousands of qubits in the near future.
In quantum communications, cryogenic engineering is essential for the operation of single-photon detectors and quantum repeaters, which are critical for secure quantum key distribution (QKD) networks. ID Quantique and Single Quantum are developing cryogenically cooled superconducting nanowire single-photon detectors (SNSPDs) that offer high detection efficiency and low noise, enabling long-distance quantum communication links. These devices are being deployed in pilot QKD networks in Europe and Asia, with further expansion expected as cryogenic reliability and integration improve.
Quantum sensing applications, such as ultra-sensitive magnetometers and gravimeters, also benefit from advances in cryogenic engineering. QuSpin and Magnicon are among the companies developing cryogenic sensors for medical imaging, geophysical exploration, and fundamental physics experiments. The next few years are expected to see broader adoption of these sensors as cryogenic systems become more compact, energy-efficient, and user-friendly.
Looking ahead, the outlook for cryogenic engineering in quantum technologies is marked by a push toward greater automation, modularity, and integration with conventional data center infrastructure. As quantum computing and communication networks scale, the industry will continue to innovate in cryogenic design, aiming to reduce operational costs and environmental impact while supporting the stringent requirements of emerging quantum applications.
Regulatory, Safety, and Standardization Initiatives (IEEE, ASME)
Cryogenic engineering is a foundational pillar for quantum computing, enabling the ultra-low temperatures required for superconducting qubits and other quantum devices. As the field matures, regulatory, safety, and standardization initiatives are becoming increasingly important to ensure safe operation, interoperability, and scalability of cryogenic systems. In 2025 and the coming years, several key organizations are shaping the landscape, notably the IEEE and the ASME.
The IEEE has been actively developing standards for quantum technologies, including those relevant to cryogenic engineering. The IEEE Quantum Initiative, launched in recent years, is working on standardizing interfaces, performance metrics, and safety protocols for quantum hardware, with a focus on cryogenic environments. In 2024, the IEEE Standards Association initiated working groups to address the unique challenges of cryogenic systems, such as electromagnetic compatibility, thermal management, and materials selection for sub-Kelvin operation. These efforts are expected to yield draft standards by 2025, providing a framework for manufacturers and research institutions to ensure compatibility and safety across quantum computing platforms.
The ASME is also playing a significant role, leveraging its expertise in pressure vessel codes and cryogenic safety. ASME’s Boiler and Pressure Vessel Code (BPVC) is being referenced and adapted for the design and certification of cryostats and dilution refrigerators used in quantum computing. In 2025, ASME is expected to release updated guidelines specifically addressing the unique operational risks of quantum cryogenics, such as rapid thermal cycling, helium management, and emergency venting procedures. These guidelines are being developed in collaboration with leading cryogenic equipment manufacturers and quantum computing companies.
Industry players such as Bluefors and Oxford Instruments—both major suppliers of dilution refrigerators—are actively participating in these standardization efforts. They are contributing practical insights from large-scale deployments in quantum computing labs and commercial installations. Their involvement ensures that emerging standards are grounded in real-world operational experience and can be rapidly adopted by the sector.
Looking ahead, regulatory and standardization activities are expected to accelerate as quantum computing moves from research to commercial deployment. The next few years will likely see the introduction of harmonized international standards, facilitating cross-border collaboration and supply chain integration. Safety protocols for handling cryogens, emergency response, and environmental considerations (such as helium conservation) will become increasingly codified, reducing operational risks and supporting the reliable scaling of quantum computing infrastructure worldwide.
Investment Landscape: Funding, M&A, and Startup Ecosystem
The investment landscape for cryogenic engineering in quantum computing has rapidly evolved as the demand for scalable, reliable, and ultra-low temperature solutions intensifies. Cryogenic systems are essential for operating superconducting and spin-based quantum processors, which require temperatures close to absolute zero. As quantum computing transitions from laboratory research to early-stage commercialization, funding and M&A activity in cryogenic engineering have accelerated, with both established players and startups attracting significant capital.
In 2024 and into 2025, major investments have flowed into companies specializing in dilution refrigerators, cryostats, and related infrastructure. Bluefors, headquartered in Finland, remains a global leader in cryogenic systems for quantum technology. The company has expanded its manufacturing capacity and R&D footprint, supported by strategic investments and partnerships with quantum hardware developers. Similarly, Oxford Instruments (UK) continues to innovate in cryogenic and superconducting technologies, leveraging its long-standing expertise to serve both academic and commercial quantum computing clients.
The startup ecosystem is vibrant, with new entrants focusing on miniaturization, automation, and energy efficiency of cryogenic platforms. Notable startups include Cryo Industries of America, which has been developing compact cryostats tailored for quantum labs, and Lake Shore Cryotronics, which is expanding its product line to address the unique needs of quantum device testing and integration. These companies have attracted seed and Series A funding rounds from both venture capital and strategic investors, reflecting confidence in the sector’s growth trajectory.
Mergers and acquisitions are also shaping the landscape. In recent years, larger instrumentation and technology firms have acquired niche cryogenics companies to vertically integrate quantum hardware supply chains. For example, Oxford Instruments has pursued targeted acquisitions to broaden its cryogenic capabilities, while Bruker Corporation has expanded its low-temperature solutions portfolio to better serve quantum research markets.
Looking ahead to 2025 and beyond, the outlook for investment in cryogenic engineering for quantum computing remains robust. The sector is expected to benefit from increased government funding for quantum initiatives in the US, EU, and Asia, as well as from the growing number of quantum computing startups requiring advanced cryogenic infrastructure. As quantum processors scale up in qubit count and complexity, demand for high-performance, reliable, and cost-effective cryogenic systems will continue to drive funding, partnerships, and consolidation in the industry.
Future Outlook: Disruptive Innovations and Long-Term Market Impact
Cryogenic engineering is a foundational enabler for quantum computing, as most leading quantum hardware platforms—such as superconducting qubits and spin qubits—require operation at temperatures near absolute zero. As the quantum computing sector matures in 2025 and beyond, disruptive innovations in cryogenic technology are poised to shape both the technical trajectory and the broader market impact of quantum systems.
A key trend is the push for scalable, modular cryogenic infrastructure. Traditional dilution refrigerators, while effective, are bulky and energy-intensive, limiting the practical deployment of large-scale quantum processors. In response, companies like Bluefors and Oxford Instruments are developing next-generation cryostats with higher cooling power, improved automation, and reduced footprint. These systems are designed to support hundreds or even thousands of qubits, addressing the anticipated scaling needs of quantum processors in the coming years.
Another area of innovation is the integration of cryogenic electronics. As quantum processors grow in complexity, the need to minimize thermal load from control wiring and electronics becomes critical. Companies such as Intel Corporation are actively researching cryo-CMOS and other low-temperature control solutions, aiming to embed classical control hardware within the cryogenic environment. This approach promises to reduce latency, improve signal integrity, and enable more compact quantum computing modules.
The market is also witnessing the emergence of specialized cryogenic component suppliers. For example, Lake Shore Cryotronics and Janis Research Company (a part of Lake Shore) are expanding their offerings of cryogenic sensors, wiring, and thermal management solutions tailored for quantum applications. These components are essential for maintaining the ultra-low temperatures and stability required for reliable quantum operations.
Looking ahead, the convergence of cryogenic engineering with quantum computing is expected to drive significant market growth and technological differentiation. As quantum hardware moves from laboratory prototypes to commercial deployment, demand for robust, scalable, and cost-effective cryogenic systems will intensify. Industry analysts anticipate that advances in cryogenic technology will not only lower the barriers to quantum adoption but also open new opportunities in adjacent fields such as quantum networking and sensing.
In summary, the next few years will likely see cryogenic engineering transition from a niche specialty to a central pillar of the quantum computing ecosystem, with disruptive innovations shaping both the pace of quantum hardware development and the structure of the emerging quantum technology market.
Sources & References
- Bluefors
- Oxford Instruments
- IBM
- Rigetti Computing
- Linde
- Cryomech Inc.
- Bluefors
- Oxford Instruments
- IBM
- Lake Shore Cryotronics, Inc.
- ID Quantique
- QuSpin
- IEEE
- ASME
- Cryo Industries of America
- Bruker Corporation
- Janis Research Company