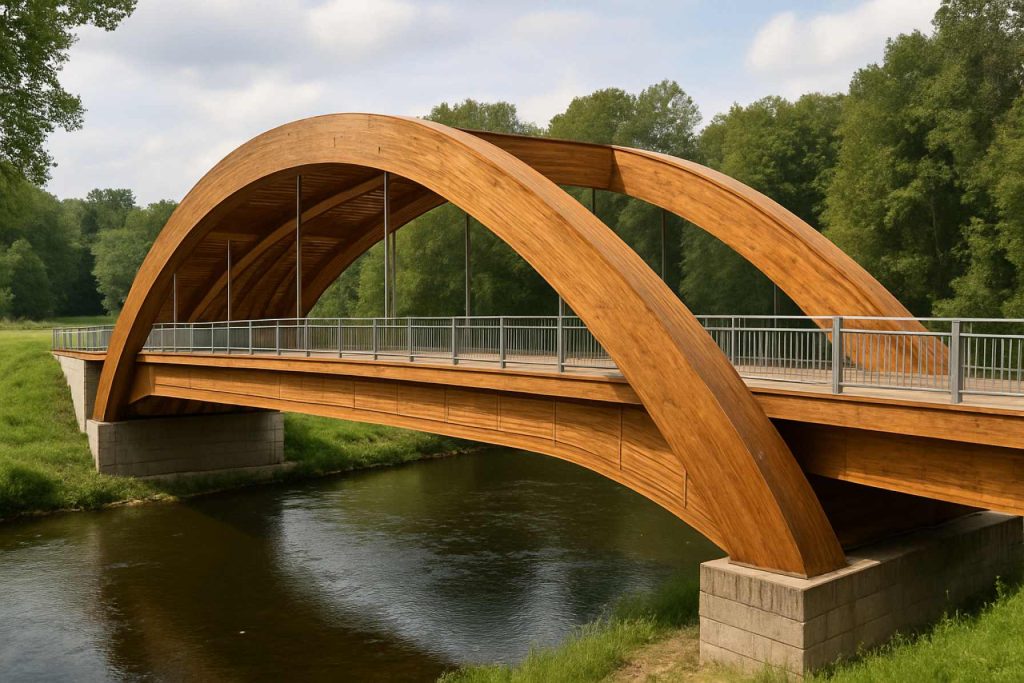
Will Orthotropic Timber Bridge Engineering Disrupt Civil Infrastructure in 2025? Discover the Surprising Innovations and Market Forecasts Shaping the Next 5 Years.
- Executive Summary: Orthotropic Timber Bridges in 2025
- Market Drivers and Growth Forecast Through 2030
- Key Players and Industry Leaders (2025 Landscape)
- Breakthrough Material Technologies and Design Innovations
- Sustainability, Carbon Footprint, and Regulatory Trends
- Project Case Studies: Successes and Lessons Learned
- Challenges: Structural Performance, Maintenance, and Costs
- Investment, Funding, and Policy Incentives
- Emerging Applications and Future Opportunities
- Strategic Outlook: Where Orthotropic Timber Bridge Engineering Is Headed by 2030
- Sources & References
Executive Summary: Orthotropic Timber Bridges in 2025
Orthotropic timber bridge engineering in 2025 stands at the convergence of sustainable construction, advanced materials science, and digital design technologies. Unlike traditional timber bridges, orthotropic designs utilize timber panels engineered with distinct properties in orthogonal directions, often combined with CLT (cross-laminated timber) or glulam (glued laminated timber), to optimize load distribution and structural efficiency. The sector has experienced renewed momentum as municipalities and infrastructure authorities seek lower-carbon alternatives to concrete and steel, while also addressing maintenance, lifecycle cost, and aesthetic integration in both rural and urban contexts.
Recent years have seen pilot projects and demonstration bridges across Europe and North America, spearheaded by innovative timber manufacturers and engineering firms. Notable among industry leaders, Stora Enso and HASSLACHER Group have expanded their engineered timber production capacities, with dedicated lines for structural elements used in bridge construction. Stora Enso, for example, supplies CLT and LVL (laminated veneer lumber) panels for infrastructure projects, emphasizing both structural reliability and traceable, certified wood sourcing. Similarly, HASSLACHER Group is actively involved in large-scale timber bridge projects, leveraging in-house R&D for product development and system certification.
Key events in 2024-2025 include the launch of several municipal bridge initiatives in Scandinavia and Germany, where orthotropic timber panels are being adopted for medium-span road and pedestrian bridges. These projects benefit from advancements in digital planning and BIM (Building Information Modeling), with firms like Sweco providing integrated engineering services to optimize prefabrication and assembly. Furthermore, timber preservation and fire safety technologies—developed by suppliers such as Koppers—are increasingly incorporated into design specifications, addressing durability concerns and regulatory requirements.
The outlook for orthotropic timber bridge engineering through 2025 and beyond is optimistic. Policy drivers, including the European Green Deal and growing regional mandates for embodied carbon reduction, are expected to accelerate adoption. Manufacturers are scaling up production of high-performance timber panels, and industry collaboration is intensifying through consortia and standardization efforts led by organizations such as Finnish Wood Research. Challenges remain in harmonizing regulations and demonstrating long-term performance, but the technology is poised for broader deployment, particularly in markets prioritizing sustainability, rapid construction, and reduced maintenance costs.
Market Drivers and Growth Forecast Through 2030
Orthotropic timber bridge engineering is anticipated to experience significant growth through 2030, propelled by a convergence of technological innovation, sustainability imperatives, and evolving infrastructure policies. The increasing adoption of engineered wood products—specifically cross-laminated timber (CLT) and glulam—in bridge applications is a central market driver. These materials offer strength-to-weight ratios comparable to steel while enabling reduced carbon footprints, aligning with global decarbonization efforts in infrastructure development.
National and regional infrastructure investment programs are directly influencing the orthotropic timber bridge sector. In Europe, for example, initiatives under the EU Green Deal and “Fit for 55” legislative package encourage the use of renewable materials in civil works, favoring timber-based bridge solutions. Similarly, North American public agencies are piloting timber bridge projects, with organizations like WoodWorks supporting technical guidance and promoting mass timber adoption in the United States and Canada.
Leading manufacturers such as Stora Enso, one of the world’s largest renewable materials companies based in Finland and Sweden, have recently expanded their engineered timber production capacity, explicitly targeting bridge and civil engineering markets. HASSLACHER Group, headquartered in Austria, is another key supplier, with a portfolio including large-format glulam and CLT components specified for orthotropic bridge decks and superstructures. Their investments in automated fabrication and digital project delivery are expected to further accelerate market adoption.
From a technological standpoint, advances in adhesive technologies and hybrid timber-steel systems are improving the durability and performance of orthotropic timber bridges, reducing lifecycle maintenance costs. Collaboration between manufacturers, such as Södra (Sweden) and research bodies, is fostering the development of standardized design codes, which is critical for scaling deployment in public infrastructure contracts.
Between 2025 and 2030, industry forecasts suggest annual growth rates for timber bridge construction could exceed 8% in core markets, with particularly strong momentum in Scandinavia, Central Europe, and select North American states. This outlook is underpinned by regulatory incentives, urban expansion, and the urgent need to replace aging concrete and steel bridges with more sustainable alternatives. As digital modeling and prefabrication become mainstream, orthotropic timber bridge engineering is poised to transform both rural and urban connectivity, with an expanding roster of suppliers and stakeholders shaping the competitive landscape through the remainder of the decade.
Key Players and Industry Leaders (2025 Landscape)
In 2025, the orthotropic timber bridge engineering sector is characterized by a collaborative landscape of innovative manufacturers, specialist engineering consultancies, advanced material suppliers, and progressive public infrastructure owners. This field, which integrates orthotropic deck design principles with engineered wood solutions, is rapidly evolving due to heightened sustainability targets and technological advancements.
A pivotal role in this landscape is played by leading engineered timber producers, especially those focused on cross-laminated timber (CLT) and glued-laminated timber (glulam) products. Stora Enso, headquartered in Finland and Sweden, is among the world’s largest suppliers of mass timber and has been actively promoting engineered wood for bridge applications. The company’s innovations in CLT and LVL (laminated veneer lumber) are setting industry benchmarks for structural performance and durability in bridge decks and superstructures. Similarly, Binderholz, based in Austria, continues to expand its engineered timber portfolio and is increasingly involved in civil infrastructure projects, emphasizing the use of orthotropic principles for enhanced load distribution.
In North America, WoodWorks – Wood Products Council functions as a vital industry resource, fostering technical guidance and supporting the adoption of advanced timber systems in bridge engineering. Their initiatives in knowledge transfer and design support are accelerating the uptake of orthotropic timber deck solutions, particularly for short- and medium-span bridges.
Engineering and construction specialists are also playing a crucial role. STRABAG SE, a major European construction company, has engaged in several high-profile timber bridge projects, integrating orthotropic timber decks with hybrid structural systems. Their expertise lies in the seamless fusion of timber with steel and concrete components to optimize performance and longevity. In the Nordic region, Sweco has contributed engineering design services for landmark timber bridges, leveraging advanced modeling and material science to implement orthotropic deck concepts.
Material science leaders such as Metsä Wood are pioneering next-generation Kerto LVL and hybrid timber elements, pushing the boundaries of what is achievable in orthotropic bridge decks. Their collaborations with public infrastructure agencies across Europe have led to demonstration projects that are expected to inform broader adoption in the coming years.
Looking ahead, the sector anticipates greater participation from both public and private stakeholders. With strengthened regulatory support for sustainable infrastructure and advances in digital design tools, the industry leaders identified above are well-positioned to shape the direction of orthotropic timber bridge engineering through 2025 and beyond.
Breakthrough Material Technologies and Design Innovations
Orthotropic timber bridge engineering is experiencing a renaissance in 2025, propelled by advances in engineered wood products, digital design methodologies, and sustainability imperatives. Orthotropic systems—where deck panels exhibit different properties along different axes—are now leveraging high-performance materials and integrated design tools to deliver longer spans, improved durability, and reduced maintenance compared to earlier timber bridge generations.
A key breakthrough in recent years is the maturation of cross-laminated timber (CLT) and glued laminated timber (glulam) as orthotropic deck materials. These engineered wood products, produced by leading manufacturers such as STEICO SE and Stora Enso, offer customizable panel geometries and enhanced stiffness-to-weight ratios. For instance, CLT panels can be manufactured with varying lamella orientation and thickness, allowing designers to optimize for primary traffic loads while minimizing deflection and vibration in secondary directions.
The application of advanced connection technologies is also enabling longer and more robust orthotropic timber spans. Companies like Peikko Group are delivering concealed steel connectors and hybrid anchoring systems specifically engineered for timber bridges, improving load transfer efficiency and resilience to fatigue. Meanwhile, the adoption of self-healing coatings and high-durability adhesives is extending the service life of timber bridge components, addressing historic concerns over moisture ingress and decay.
Digitalization is accelerating orthotropic timber design in 2025. Structural modeling software, such as BIM-integrated platforms and parametric engineering tools, is being widely used to simulate orthotropic behavior, optimize material usage, and generate complex geometries for prefabrication. This approach is endorsed by industry organizations including WoodWorks, which provides technical guidance and case studies on orthotropic timber applications in bridge projects.
Looking ahead, the outlook for orthotropic timber bridges is strongly positive. Public infrastructure initiatives in Europe and North America are increasingly favoring renewable materials with low embodied carbon, with timber bridges gaining traction as sustainable alternatives to steel or concrete spans. As regulatory standards evolve and supply chains mature, leading suppliers such as Binderholz GmbH and Metsä Wood are expanding production capacities for large-format orthotropic panels and custom bridge kits. Analysts anticipate a steady increase in orthotropic timber bridge deployments, particularly in rural and semi-urban contexts where rapid installation and reduced environmental impact are critical.
Sustainability, Carbon Footprint, and Regulatory Trends
Orthotropic timber bridge engineering is experiencing renewed attention as sustainability becomes a central criterion in infrastructure development. The unique orthotropic properties of engineered timber—where material strength varies directionally—allow for efficient designs that minimize material use while maximizing structural performance. In 2025 and the next few years, this efficiency aligns with global and regional regulatory pushes toward low-carbon, environmentally responsible construction.
The carbon footprint of bridge construction is under intense scrutiny, with governments and industry bodies mandating reductions in embodied carbon. Timber, as a renewable resource and carbon sink, offers distinct advantages over traditional steel and concrete. Life-cycle assessments (LCA) consistently show that engineered wood bridges capture and store atmospheric CO2 during growth and retain it throughout the bridge’s service life, offsetting emissions from manufacture and transport. Organizations such as PEFC and FSC offer certification schemes to ensure timber comes from sustainably managed forests, which is increasingly required in public procurement and infrastructure tenders.
On the regulatory front, the European Union’s Green Public Procurement criteria and the 2025 updates to the Construction Products Regulation (CPR) are expected to further incentivize the use of renewable, traceable materials in civil works. In North America, the Western Wood Products Association and WoodWorks are actively promoting best practices and compliance with updated codes, such as the American Association of State Highway and Transportation Officials (AASHTO) LRFD Bridge Design Specifications, which are increasingly recognizing engineered wood and hybrid timber solutions.
Manufacturers like KLH Massivholz and Stora Enso are scaling up production of cross-laminated timber (CLT) and glued laminated timber (glulam) components that meet rigorous structural and environmental standards. These materials are integral to orthotropic bridge designs that optimize load paths and durability, while facilitating recyclability or repurposing at end-of-life. Pilot projects in Scandinavia, Canada, and Central Europe are demonstrating real-world performance, supported by monitoring data and third-party environmental product declarations (EPDs).
Looking forward, sustainability reporting—including carbon footprint disclosure—will be mandatory for an increasing number of infrastructure projects, driven by both regulation and investor expectations. As a result, orthotropic timber bridge engineering is poised for accelerated adoption, provided that industry stakeholders maintain transparency in sourcing and performance, and continue to innovate in design and lifecycle management.
Project Case Studies: Successes and Lessons Learned
In 2025, orthotropic timber bridge engineering continues to gain traction worldwide, driven by sustainability goals, advances in engineered wood products, and the need for rapid, lightweight construction. Recent project case studies highlight both the successes and the critical lessons learned in this evolving sector.
One landmark project is the Vihantasalmi Bridge in Finland, which utilizes an orthotropic deck system made from glued laminated timber (glulam) and cross-laminated timber (CLT). This bridge, maintained by Stora Enso—a global leader in renewable materials—demonstrates high load-carrying capacity and effective resistance to dynamic loads, thanks to the orthotropic action of the timber panels. The project’s success underscores the importance of precise moisture control during fabrication and installation, as excessive moisture variations were found to impact long-term performance.
In North America, StructureCraft, a Canadian engineering and fabrication company specializing in timber structures, has led several orthotropic bridge projects. Their work on the Fort St. John Pedestrian Bridge in British Columbia, completed in late 2024, showcased rapid modular assembly and minimized site disruption. StructureCraft’s use of dowel-laminated timber (DLT) for the orthotropic deck achieved significant durability and reduced maintenance costs. However, the project emphasized the need for detailed connection design, as early prototypes suffered from connector fatigue under cyclic loading.
In Central Europe, HASSLACHER Group has been instrumental in promoting orthotropic timber bridge systems. Their involvement in the 2023 reconstruction of the Murtal Bridge in Austria, using custom-milled glulam panels, demonstrated that prefabrication is key to both quality control and speed of installation. Nonetheless, the project revealed that long-term monitoring of moisture ingress at deck joints remains crucial, as even minor lapses can lead to localized degradation.
Emerging lessons learned across these case studies include the necessity for robust waterproofing detailing, the integration of advanced sensors for structural health monitoring, and the value of early collaboration between timber suppliers, engineers, and contractors. Looking ahead to the next few years, industry leaders such as Stora Enso, StructureCraft, and HASSLACHER Group are likely to expand their portfolio of orthotropic timber bridges, leveraging digital design tools and improved connections to address remaining durability challenges. The sector is set to benefit from increasing governmental support for low-carbon infrastructure, further accelerating adoption and innovation in orthotropic timber bridge engineering.
Challenges: Structural Performance, Maintenance, and Costs
Orthotropic timber bridge engineering in 2025 faces a complex array of challenges related to structural performance, maintenance requirements, and overall lifecycle costs. Although the adoption of orthotropic timber decks and systems—utilizing engineered wood products such as glulam and cross-laminated timber (CLT)—has accelerated in response to sustainability goals, several technical and economic hurdles remain.
A primary concern is the long-term structural performance of timber components under variable loads and environmental exposure. While modern engineered timbers offer enhanced strength-to-weight ratios and dimensional stability, they are inherently sensitive to moisture ingress, biological attack, and ultraviolet (UV) degradation. Recent bridge projects in Europe and North America have demonstrated that, despite advanced protective coatings and detailing, periodic inspection and maintenance are indispensable to mitigate decay and ensure load-bearing capacity over the intended service life.
Maintenance regimes for orthotropic timber bridges tend to be more intensive than those for steel or concrete alternatives. Moisture monitoring, surface treatment renewal, and joint resealing are cited by manufacturers as critical activities, especially in regions with freeze-thaw cycles or high humidity. Companies like Stora Enso—a major supplier of CLT and glulam—explicitly recommend tailored inspection and maintenance schedules to preserve structural integrity and aesthetic quality. Similarly, Binderholz, another leading engineered timber producer, has invested in research to improve durability and minimize maintenance demands through factory-applied treatments and design innovations.
Cost remains a contentious issue. Initial construction costs for orthotropic timber bridges can be competitive due to prefabrication efficiencies and lighter superstructures, reducing foundation requirements. However, whole-life cost calculations must factor in more frequent maintenance cycles and the potential need for partial component replacement. According to suppliers, the cost-benefit equation can shift favorably in regions with ready access to sustainably sourced timber and skilled labor, but less so where these are lacking or where harsh environmental exposures prevail.
Looking ahead to the next few years, industry bodies like WoodWorks anticipate incremental improvements in structural adhesives, connection systems, and hybrid timber-concrete designs, which may alleviate some performance and maintenance challenges. Digital monitoring and predictive maintenance technologies, being piloted by manufacturers and bridge operators, promise to optimize inspection intervals and reduce lifecycle costs by enabling proactive interventions. Nonetheless, widespread adoption of orthotropic timber bridge engineering will likely depend on continued technical innovation, demonstration projects, and the maturation of standards that address persistent concerns regarding durability, safety, and cost effectiveness.
Investment, Funding, and Policy Incentives
Orthotropic timber bridge engineering is experiencing renewed investment and policy attention in 2025, driven by the global shift toward sustainable infrastructure and decarbonization goals. Major public and private funding initiatives are being channeled toward research, pilot projects, and full-scale deployment, particularly in Europe, North America, and parts of Asia-Pacific.
In the European Union, the European Union continues to allocate funds through its Green Deal initiatives and Horizon Europe research programs, supporting timber infrastructure that contributes to reduced embodied carbon. Notably, Scandinavian countries have increased direct investment in timber bridge demonstration projects, leveraging their advanced forestry and timber manufacturing sectors. National and regional transport agencies, such as Sweden’s Trafikverket, are actively piloting large-span orthotropic timber bridges, utilizing public procurement incentives to encourage market adoption.
In North America, Canada’s Natural Resources Canada and provincial ministries have expanded grant programs to advance engineered wood products in infrastructure, including orthotropic bridge designs. The U.S. Department of Agriculture, through its Forest Service Wood Innovations Grants, continues to provide funding for mass timber bridge prototypes and research, aligning with federal Buy Clean and sustainable procurement policies. States with strong forestry economies, such as Oregon and Washington, are leveraging these incentives to stimulate local supply chains and bridge construction.
Private sector investment is also growing, with engineered timber manufacturers such as Stora Enso and Binderholz supporting R&D collaborations and demonstration projects. These companies are expanding production capacity for cross-laminated timber (CLT) and glulam panels suitable for orthotropic bridge applications. Their involvement is often matched by public co-funding, reflecting confidence in the commercial scalability of these technologies.
Policy incentives in 2025 focus on streamlined permitting, fast-track approvals for low-carbon construction, and preferential scoring for timber solutions in public tenders. Several countries are revising bridge design codes to explicitly address orthotropic timber systems, reducing regulatory uncertainty. Additionally, voluntary certification programs—such as those promoted by the Programme for the Endorsement of Forest Certification—are being linked with procurement criteria to ensure the sustainability of wood sourcing.
Looking ahead, these investment, funding, and policy mechanisms are expected to accelerate the mainstreaming of orthotropic timber bridge engineering over the next several years. Ongoing monitoring of lifecycle performance and cost-effectiveness will be critical to maintaining policy and market support, with the results of current pilot projects likely to influence future funding allocations and regulatory frameworks.
Emerging Applications and Future Opportunities
Orthotropic timber bridge engineering is entering a transformative period, with 2025 marking a notable acceleration in both technical innovation and practical applications worldwide. The push for sustainable infrastructure, advances in engineered wood products, and a growing number of successful pilot projects are creating new momentum for orthotropic timber solutions in bridge design and construction.
Recent years have seen the deployment of orthotropic timber decks and superstructures in pedestrian, cycling, and light vehicle bridges, especially in regions with strong commitments to carbon reduction. For example, Scandinavian countries, Austria, and Switzerland have demonstrated the performance and durability of cross-laminated timber (CLT) and glue-laminated timber (glulam) in orthotropic bridge systems, often in hybrid configurations with steel or concrete elements. Companies such as Binderholz and Stora Enso—both major suppliers of mass timber—are actively supporting bridge projects with advanced timber products and engineering expertise, aiming to optimize load distribution and fatigue resistance in orthotropic configurations.
Emerging applications in 2025 are focusing on modular construction and rapid assembly, with orthotropic timber panels being prefabricated offsite for efficient installation. This approach is particularly advantageous for urban infrastructure upgrades, where bridge downtime must be minimized. Recent pilot projects have demonstrated that orthotropic timber decks can reduce installation times by up to 50% compared to traditional concrete decks, while maintaining competitive life-cycle costs. Research collaborations between industry and academic partners are also enhancing digital design tools and predictive maintenance models, further improving the reliability and scalability of these solutions.
Outlook for the next few years indicates a growing role for orthotropic timber bridges in rural road networks, ecological corridors, and environmentally sensitive areas. Policy incentives for low-carbon construction materials, such as those implemented by the European Union and certain US states, are expected to drive further adoption. Leading industry players—including Wiehag, recognized for their expertise in large-span engineered timber structures, and Kebony, known for their modified wood products with enhanced durability—are positioning themselves at the forefront of this growth, expanding production and R&D to support larger and more complex bridge applications.
In summary, 2025 is set to be a pivotal year for orthotropic timber bridge engineering, with new applications, improved performance data, and expanding industry capabilities paving the way for broader adoption and increasingly ambitious projects in the near future.
Strategic Outlook: Where Orthotropic Timber Bridge Engineering Is Headed by 2030
Orthotropic timber bridge engineering is positioned at a transformative juncture as we enter 2025, driven by advances in engineered wood products, sustainability imperatives, and the integration of digital design and monitoring technologies. The sector has seen a marked increase in the adoption of orthotropic deck systems—where timber panels are engineered with distinct properties in orthogonal directions to optimize load distribution and structural efficiency. This trend aligns with global initiatives to reduce embodied carbon in infrastructure, with timber bridges increasingly recognized for their low carbon footprint compared to steel and concrete alternatives.
Major European timber product manufacturers such as Stora Enso and Hasslacher Group are investing in high-performance cross-laminated timber (CLT) and glulam solutions, tailored for bridge applications. These companies are collaborating with leading civil engineering firms to develop next-generation orthotropic timber decks with enhanced durability and modularity. For example, Stora Enso offers bridge-specific CLT panels engineered for rapid installation and optimized for longevity, while Hasslacher Group is supplying glulam elements for hybrid bridge projects across Europe.
In North America, initiatives supported by bodies such as WoodWorks and American Wood Council are driving standardization and knowledge transfer to encourage broader adoption of orthotropic timber systems in state and municipal bridge programs. The development of updated design guidelines and codes is anticipated by 2027, which is expected to streamline permitting processes and catalyze further market uptake.
Digitalization is another key driver. Structural health monitoring (SHM) using embedded sensors and digital twins is being piloted in timber bridge projects, especially in Scandinavia and Central Europe. Companies such as Stora Enso and design partners are leveraging Building Information Modeling (BIM) and Internet of Things (IoT) platforms to provide real-time performance data, facilitating predictive maintenance and life-cycle optimization.
By 2030, experts expect orthotropic timber bridge engineering to be defined by:
- Widespread use of modular, prefabricated orthotropic timber deck systems in both urban and rural bridge construction.
- Integration of advanced wood protection technologies, extending service life and reducing maintenance costs.
- Greater collaboration between industry leaders (such as Hasslacher Group and Stora Enso) and public infrastructure agencies.
- New standards and performance-based codes, enabling larger spans and more complex applications.
With robust supply chains, continued R&D investment, and policy support for sustainable infrastructure, orthotropic timber bridge engineering is set for significant growth and innovation by the end of the decade.
Sources & References
- HASSLACHER Group
- Sweco
- Koppers
- WoodWorks
- Södra
- Binderholz
- STRABAG SE
- Sweco
- Metsä Wood
- STEICO SE
- Peikko Group
- PEFC
- FSC
- KLH Massivholz
- StructureCraft
- European Union
- Natural Resources Canada
- Binderholz
- Programme for the Endorsement of Forest Certification
- Wiehag