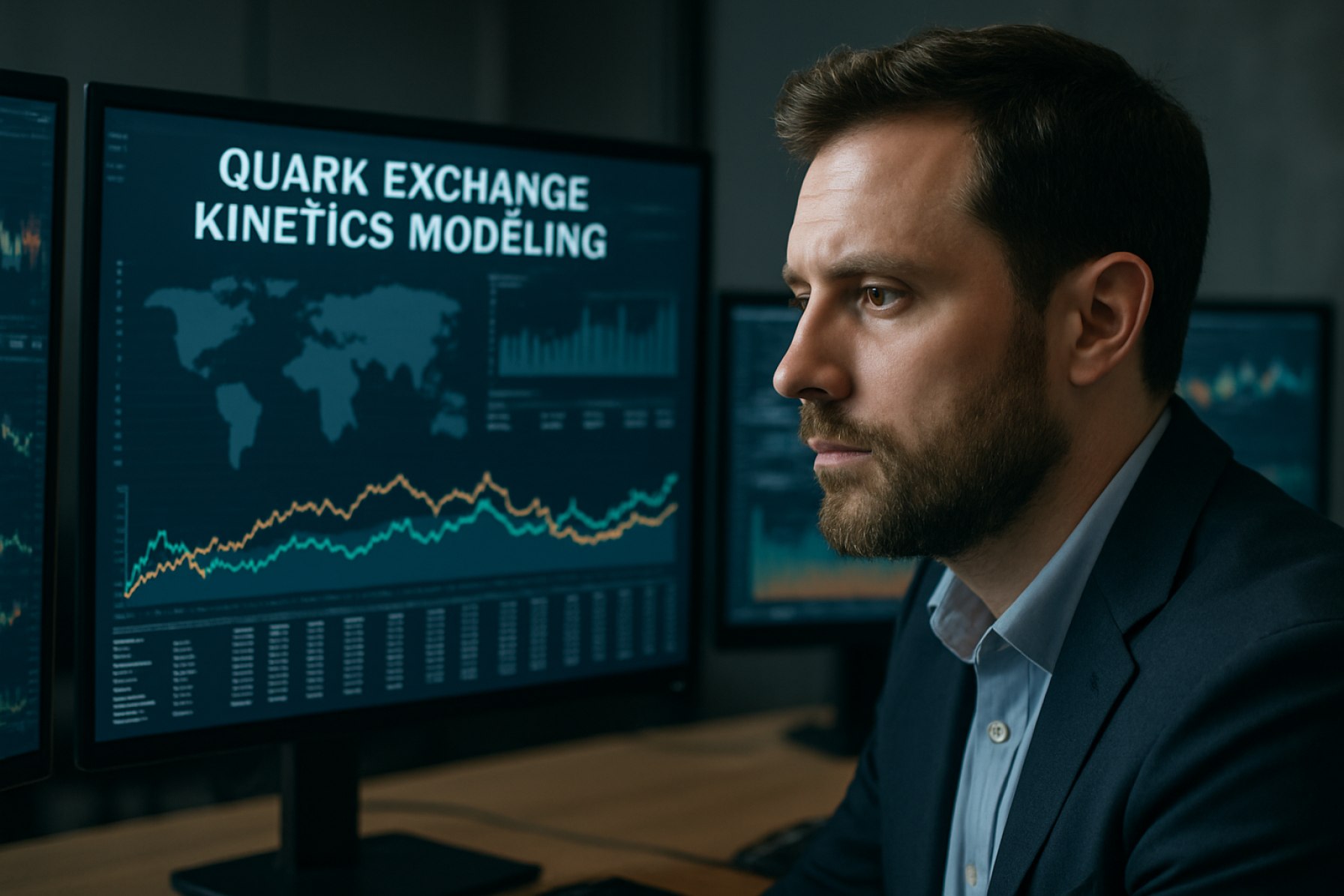
Table of Contents
- Executive Summary: 2025 Market Pulse and Strategic Highlights
- Technology Foundations: Principles of Quark Exchange Kinetics Modeling
- Key Players & Industry Ecosystem (2025)
- Recent Breakthroughs Transforming Modeling Accuracy
- Emerging Applications: Quantum Computing, Particle Physics, and Beyond
- Competitive Landscape: Leading Innovators & Strategic Alliances
- Market Forecast: Growth Projections Through 2030
- Challenges & Barriers: Technical, Regulatory, and Scalability Issues
- Opportunities & Investment Hotspots (2025–2030)
- Future Outlook: Next-Gen Modeling, Collaboration, and Disruptive Potential
- Sources & References
Executive Summary: 2025 Market Pulse and Strategic Highlights
The year 2025 marks a pivotal period for the advancement of quark exchange kinetics modeling, as quantum chromodynamics (QCD) simulations and experimental validation accelerate across global research institutions and specialized technology suppliers. Key developments are being driven by the convergence of high-performance computing, novel quantum algorithms, and collaborative international research frameworks. The increasing accessibility of exascale supercomputing resources, notably from entities such as IBM and Hewlett Packard Enterprise, is enabling more precise and large-scale kinetic modeling of quark interactions within hadronic matter. This computational leap is fostering rapid iteration cycles and enhanced predictive accuracy for both fundamental science and applied fields, including advanced materials and nuclear technology.
Throughout 2025, the sector is witnessing a shift from purely theoretical constructs to hybridized experimentation, spearheaded by collaborations linking academic institutions, national laboratories, and industry. Notably, several international research consortia—including those supported by CERN and Brookhaven National Laboratory—are integrating real-time data from particle accelerators with next-generation modeling tools. These efforts are yielding unprecedented insights into the time-dependent exchange dynamics of quarks, gluon flux tubes, and color confinement phenomena. Direct experimental feedback is now being used to calibrate and validate kinetic models, closing the gap between simulation and observation.
Market traction is further indicated by expanding industry interest in quantum computational solutions for QCD, with leading hardware providers such as Intel and NVIDIA developing dedicated processor architectures optimized for complex particle simulations. The emergence of specialized software platforms—often in collaboration with academic groups—has prompted a new wave of commercial tools tailored for both research and industrial users. These platforms are streamlining the workflow for modeling quark exchange processes, reducing computational overhead, and enabling broader adoption beyond traditional physics domains.
Looking ahead, the outlook for quark exchange kinetics modeling in the next several years is robust. As hardware and algorithmic innovations continue to mature, the field is expected to experience further democratization, with cloud-based modeling services and open-source frameworks lowering barriers to entry. Strategic partnerships between technology firms, research institutions, and government stakeholders will be central to sustaining momentum. The integration of real-time experimental data into kinetic models is poised to unlock new applications in materials science, energy generation, and quantum information processing, positioning quark exchange kinetics modeling as a critical enabler of next-generation scientific and industrial breakthroughs.
Technology Foundations: Principles of Quark Exchange Kinetics Modeling
Quark Exchange Kinetics Modeling represents a rapidly advancing field at the intersection of particle physics, computational modeling, and high-performance simulation. The core principle underlying this domain is the quantification and prediction of quark exchange processes—fundamental interactions that govern the structure and transformation of hadrons under various energy regimes. In 2025, the technology foundation for quark exchange modeling is built upon quantum chromodynamics (QCD), the fundamental theory describing strong interactions among quarks and gluons. Modern modeling efforts utilize lattice QCD computations, Monte Carlo simulation frameworks, and machine learning–driven parameter optimizations, all of which are increasingly enabled by advances in supercomputing architectures.
At the hardware level, significant strides in exascale computing are accelerating the fidelity and scope of kinetic models. The deployment of exascale systems such as those at Oak Ridge National Laboratory and Argonne National Laboratory allows researchers to simulate multi-quark exchange kinetics with greater spatial and temporal resolution, incorporating complex phenomena such as color confinement, sea quark fluctuations, and emergent collective behaviors. These computational resources are complemented by advancements in GPU-accelerated computing, which have been harnessed in frameworks like the MILC code and the Chroma software suite used by collaborations at institutions like Fermi National Accelerator Laboratory.
Recent data from high-energy collider experiments, notably those at CERN (Large Hadron Collider) and Brookhaven National Laboratory (Relativistic Heavy Ion Collider), are providing critical benchmarks for validating and refining quark exchange models. These experiments yield vast datasets on hadronization patterns, multi-parton interactions, and exotic state formation, all of which are directly informing the parameter spaces and validation protocols of kinetic models. Such empirical feedback loops are essential for improving the predictive power of simulation tools.
Looking ahead, the field is poised for transformative progress as new experimental facilities—such as the Electron-Ion Collider (EIC) under construction at Brookhaven—come online. These platforms will enable unprecedented exploration of quark-gluon dynamics and the detailed mapping of exchange processes. Simultaneously, collaborations with quantum computing initiatives, including those supported by IBM and Intel, hold promise for tackling the exponentially complex state spaces inherent in quark kinetic modeling. Over the next several years, the convergence of experimental data, advanced algorithms, and scalable computation is expected to drive significant breakthroughs in both foundational understanding and applied modeling capabilities.
Key Players & Industry Ecosystem (2025)
The field of Quark Exchange Kinetics Modeling stands at a pivotal juncture in 2025, shaped by the convergence of high-energy physics research, advanced computational platforms, and collaborative international projects. The ecosystem is defined by a tightly interwoven network of research laboratories, academic institutions, and technology providers, each playing a unique role in advancing both the theoretical and practical frontiers of quark-level dynamics.
Key players include major particle physics laboratories spearheading experimental and computational efforts in quark exchange phenomena. CERN remains at the forefront, utilizing its Large Hadron Collider (LHC) experiments—such as ATLAS and CMS—to generate and analyze data critical for validating and refining quark exchange kinetic models. These collaborations have recently intensified efforts to probe multi-quark states and rare exchange processes, leveraging upgraded detector systems and enhanced data acquisition rates. Similarly, Fermi National Accelerator Laboratory (Fermilab) continues to contribute through its ongoing work in heavy flavor physics and lattice quantum chromodynamics (QCD) simulations, underpinning many kinetic modeling breakthroughs.
On the computational side, organizations like NVIDIA Corporation and IBM are increasingly influential, providing high-performance computing (HPC) architectures and AI-accelerated platforms vital for running complex QCD simulations and real-time event reconstruction. In close partnership with leading research facilities, these companies facilitate the scaling of kinetic models to handle the vast amounts of data generated in modern collider experiments.
Japan’s High Energy Accelerator Research Organization (KEK) and Germany’s Deutsches Elektronen-Synchrotron (DESY) are active contributors through their respective accelerator programs and collaborative initiatives in theoretical modeling. Both institutions are engaged in international consortia focusing on open data standards and interoperable modeling frameworks, which are increasingly seen as essential for accelerating progress and ensuring reproducibility in quark exchange kinetics.
Looking ahead, the industry ecosystem is expected to see deeper integration between experimental physics, AI-driven modeling, and cloud-based data sharing platforms. Initiatives aimed at open science and community-driven software—such as those fostered by CERN and global partners—will likely further democratize access to modeling tools and datasets. As detector upgrades and computational power continue to scale, the next few years are poised to yield more precise, predictive models of quark exchange kinetics, supporting both fundamental research and emerging quantum technologies.
Recent Breakthroughs Transforming Modeling Accuracy
The landscape of quark exchange kinetics modeling has witnessed transformative breakthroughs in recent years, driven by advances in computational power, algorithmic innovation, and collaborative international research. In 2025, several milestones have significantly elevated the accuracy and predictive capability of models describing the dynamic exchange of quarks in high-energy physics environments.
One of the most impactful developments has been the integration of machine learning techniques with traditional quantum chromodynamics (QCD) simulations. Researchers at major particle physics laboratories, such as European Organization for Nuclear Research (CERN) and Brookhaven National Laboratory, have reported the successful deployment of deep neural networks to accelerate the computation of color flow and multi-quark interactions. These approaches have enabled the simulation of complex quark exchange processes within femtoscopic timescales, which was previously computationally prohibitive.
Additionally, the adoption of exascale computing platforms has allowed for unprecedented resolution in lattice QCD calculations. Facilities at Lawrence Livermore National Laboratory and Thomas Jefferson National Accelerator Facility have demonstrated the capacity to resolve subtle kinetic phenomena, such as diquark correlations and transient multi-body exchange events, with much higher fidelity. These advances are directly contributing to more accurate modeling of hadronization and the internal structure of baryons and mesons.
In 2024 and into 2025, collaborative projects between experimental and theoretical groups have provided crucial validation of model predictions. For instance, data from the Large Hadron Collider’s Run 3, managed by CERN, has yielded new insights into the frequency and distribution of quark exchange events during heavy-ion collisions. The synergy between experimental measurements and real-time simulation feedback is closing the gap between theoretical models and observed particle behavior.
Looking ahead, the implementation of quantum computing frameworks promises to further revolutionize quark exchange kinetics modeling. Initiatives at IBM and partnerships with major physics institutions aim to leverage quantum algorithms to tackle the combinatorial complexity of multi-quark systems. If realized, these efforts could drastically reduce simulation times while enhancing predictive accuracy.
Collectively, these breakthroughs are not only refining fundamental understanding but are also laying the groundwork for new discoveries in particle and nuclear physics over the next several years, as the interplay of advanced computation and high-precision experimentation continues to drive the field forward.
Emerging Applications: Quantum Computing, Particle Physics, and Beyond
Quark exchange kinetics modeling is rapidly evolving into a pivotal computational tool bridging the frontiers of quantum computing and particle physics. As of 2025, the intense focus on accurately simulating quark-level interactions—essential for understanding phenomena such as color confinement and hadronization in quantum chromodynamics (QCD)—is driving new development trajectories in both theoretical frameworks and practical applications.
In particle physics, large-scale experiments like those at CERN are generating unprecedented amounts of collision data, particularly from the Large Hadron Collider (LHC). These data sets are fueling demand for advanced modeling of quark exchange kinetics to interpret complex multi-particle events and to refine theoretical predictions. Efforts are underway to integrate quark exchange models into broader QCD event generators, allowing for more precise tuning against experimental results. For example, collaborations within CERN and other global research infrastructures continue to refine lattice QCD algorithms and stochastic modeling approaches to better capture non-perturbative quark dynamics.
On the quantum computing front, companies such as IBM and Intel are actively exploring quantum algorithms tailored for simulating QCD processes, including quark exchange kinetics. These initiatives are propelled by the recognition that conventional supercomputing, while powerful, faces scalability bottlenecks as model dimensionality increases. Quantum hardware advances, anticipated to accelerate through 2025 and beyond, are expected to improve the fidelity and scale of such simulations, potentially enabling real-time exploration of quark-gluon plasma evolution and other high-energy phenomena.
Emerging interdisciplinary collaborations, such as those fostered by Brookhaven National Laboratory, are leveraging machine learning in tandem with quantum and classical simulations to optimize parameter estimation in quark exchange models. These hybrid approaches are already demonstrating promise in extracting new physics from noisy or incomplete data—a critical capability as experiments reach deeper into uncharted energy regimes.
Looking ahead, the outlook for quark exchange kinetics modeling is strongly positive. The convergence of quantum hardware innovation, algorithmic breakthroughs, and high-fidelity experimental data is expected to yield richer, more predictive models. Anticipated milestones for the next few years include the first demonstration of quantum advantage in simulating non-trivial QCD systems and the deployment of real-time kinetic modeling in support of next-generation collider experiments. As global investment in quantum and high-energy physics infrastructure continues, quark exchange kinetics modeling will remain a focal point for both fundamental discovery and emerging technological applications.
Competitive Landscape: Leading Innovators & Strategic Alliances
The competitive landscape for quark exchange kinetics modeling has intensified through 2025, driven by a combination of theoretical advancements, high-performance computing (HPC), and international collaborations. The field, central to understanding the dynamics of quarks within hadrons and nuclear matter, is predominantly shaped by research institutions, national laboratories, and a select group of computing hardware suppliers.
Key innovation is occurring at major research centers such as Brookhaven National Laboratory and CERN, both of which continue to invest in simulation software and data analysis platforms. At Brookhaven, the Relativistic Heavy Ion Collider (RHIC) has enabled high-precision measurements that inform the calibration and validation of quark exchange kinetic models, with ongoing upgrades slated to further enhance data fidelity through 2026. CERN’s Large Hadron Collider (LHC) experiments, particularly ALICE, also provide vast datasets on quark-gluon plasma formation and hadronization, which are being leveraged to refine exchange kinetics at the sub-femtometer scale.
Strategic alliances are a hallmark of the sector. The U.S. Department of Energy’s Exascale Computing Project, involving Oak Ridge National Laboratory and others, is working with academic groups to port quantum chromodynamics (QCD) codes to next-generation supercomputers. These codes are fundamental to simulating quark exchange processes with higher accuracy and at greater scales. The synergy between software developers and HPC hardware suppliers—such as NVIDIA and Intel—is critical, as the latest GPUs and CPUs are tailored for the complex lattice QCD calculations required in these models.
Japan’s RIKEN institute, working through the “K computer” and its successors, maintains a leadership role in lattice QCD, with frequent collaboration with European and American partners to benchmark and cross-validate quark exchange kinetic models across diverse hardware and algorithmic approaches. The European Organization for Nuclear Research, via its open data initiatives, further facilitates code sharing and validation by the global theoretical physics community.
Looking ahead, the launch of the Electron-Ion Collider (EIC) at Brookhaven is expected to act as a catalyst for new alliances and rapid evolution in modeling techniques. This facility will generate unprecedented experimental data on nucleon structure and quark-gluon interactions, offering new benchmarks for kinetic models. The convergence of experimental capability, open-source software development, and next-generation HPC is likely to deepen collaborations among national labs, universities, and hardware manufacturers, shaping a competitive but highly collaborative landscape through 2027 and beyond.
Market Forecast: Growth Projections Through 2030
The market for Quark Exchange Kinetics Modeling is entering a pivotal growth phase in 2025, driven by surging interest in high-fidelity quantum simulations and the need for accurate modeling of subatomic particle interactions. The increasing computational capabilities of quantum hardware and advanced classical supercomputers are enabling more detailed and large-scale simulations, which were previously unattainable. This is particularly relevant for sectors such as fundamental particle physics, quantum materials research, and next-generation quantum computing architectures.
In the current year, leading research institutions and technology companies are expanding initiatives in quantum simulation platforms that facilitate quark-level modeling. For example, major advancements in programmable quantum devices have been reported by IBM and Intel, both of which are developing hardware and algorithms targeted at simulating quantum chromodynamics (QCD) and related phenomena. These efforts are closely aligned with collaborations between industry and major research consortia, such as those coordinated by CERN and Brookhaven National Laboratory (BNL), both of which have demonstrated a commitment to advancing QCD simulation frameworks and integrating kinetics modeling into larger-scale experiments.
From a market perspective, the immediate outlook (2025-2027) is characterized by increased R&D investments, pilot deployments, and cross-disciplinary projects that leverage both AI-accelerated classical computing and quantum hardware. The fusion of machine learning-driven parameter optimization with quantum simulation is expected to enhance the predictive accuracy of quark exchange kinetics models, further encouraging adoption across academic laboratories, national research facilities, and, to a lesser extent, private-sector R&D centers. Industry bodies such as IEEE are also establishing standards for simulation protocols and data interoperability, which will support broader ecosystem growth over the forecast period.
By 2030, the Quark Exchange Kinetics Modeling sector is projected to experience robust expansion, underpinned by ongoing hardware improvements and the maturation of hybrid quantum-classical algorithms. The entry of additional players from the semiconductor and high-performance computing domains is anticipated, with companies like NVIDIA and AMD poised to contribute GPU-accelerated solutions tailored for particle interaction modeling. With fundamental discoveries and commercial applications on the horizon, the field is expected to remain on a strong upward trajectory, supported by continued collaboration among technology providers, research organizations, and standards bodies.
Challenges & Barriers: Technical, Regulatory, and Scalability Issues
Quark exchange kinetics modeling, a cornerstone in advancing quantum chromodynamics (QCD) applications and high-energy particle interaction simulations, faces several formidable challenges as of 2025. These challenges span technical complexities, regulatory ambiguities, and scalability bottlenecks that must be addressed to enable broader adoption and impactful scientific breakthroughs.
Technically, the non-perturbative nature of QCD remains a primary obstacle. Modeling the exchange of quarks—particularly in multi-body systems—demands immense computational resources due to the complex calculations involved in lattice QCD and effective field theory approaches. Even with ongoing advancements in supercomputing infrastructure, such as those developed by IBM and NVIDIA, the sheer scale of the data and the need for real-time or near-real-time modeling introduce latency and memory bandwidth limitations. Furthermore, accurately simulating confinement and color charge dynamics at femtometer scales is still hindered by limitations in both algorithmic efficiency and hardware capabilities.
From a regulatory perspective, the lack of established standards for data integrity, model validation, and reproducibility in high-energy physics modeling is a continuing concern. Organizations such as CERN and Brookhaven National Laboratory are working to define best practices, but there remains no unified framework for cross-platform validation or for the ethical use of advanced modeling, particularly as AI-driven methods become integrated with traditional physics simulations. Regulatory gaps also exist around intellectual property for custom-developed algorithms and the sharing of high-value simulation data across borders—issues that are only beginning to be addressed through international collaboration.
Scalability is another significant barrier. The transition from small-scale academic testbeds to large-scale, production-level applications in experimental facilities is impeded by both software and hardware constraints. For instance, integrating novel quantum computing solutions from entities like IBM or leveraging GPU-accelerated platforms from NVIDIA requires substantial adaptation of legacy codes and the development of new interoperability protocols. Moreover, the high operational costs and energy demands of maintaining cutting-edge simulation clusters present economic and sustainability challenges for research institutions.
Looking ahead, the sector is expected to see incremental progress on each of these fronts, driven by collaborations between major research facilities, hardware vendors, and policy-setting bodies. However, the pace of adoption and impact on experimental QCD research will remain closely tied to breakthroughs in computational power, algorithmic innovation, and the establishment of robust regulatory frameworks.
Opportunities & Investment Hotspots (2025–2030)
The landscape for quark exchange kinetics modeling is evolving rapidly as both fundamental research and applied technology sectors recognize its potential. Between 2025 and 2030, several significant investment and partnership opportunities are expected to emerge, driven by advances in quantum computing, high-performance simulation platforms, and deepening collaborations between academia and industry.
One of the primary opportunities lies in the integration of quark exchange kinetics models with next-generation quantum computing infrastructure. Quantum simulation capabilities are being actively developed by leading technology companies, which will allow for more realistic and computationally feasible modeling of subatomic particle interactions. For instance, organizations like IBM and Intel Corporation are investing in quantum hardware and software ecosystems that can support such high-fidelity simulations, potentially enabling breakthroughs in both materials science and high-energy physics.
Furthermore, the coming years will likely see increased funding and collaboration opportunities from national laboratories and particle physics consortia. Agencies such as CERN are expected to expand their computational physics programs, offering partnership grants and co-development projects targeting the refinement and application of kinetic models for quark-gluon plasma, nucleon structure, and beyond. These collaborative frameworks are particularly attractive for start-ups or research groups specializing in algorithmic innovation or data-driven modeling techniques.
On the commercial front, the maturation of kinetic modeling tools is expected to open up licensing and service opportunities, especially as industries such as advanced manufacturing, aerospace, and defense seek to harness fundamental particle dynamics for novel material and energy solutions. Companies actively developing simulation software, such as Ansys, Inc., may move to incorporate quark-level modeling modules into their multiphysics platforms, creating new markets for specialized computational toolkits.
Looking ahead, the convergence of high-speed data acquisition from experimental facilities—for example, the upgrades at the Brookhaven National Laboratory—with AI-enhanced modeling frameworks presents a fertile ground for investment in hybrid data-simulation pipelines. These systems can accelerate the iterative refinement of kinetic models, reducing the gap between theory and experimental validation.
Overall, the period from 2025 to 2030 is poised to be a dynamic phase for quark exchange kinetics modeling, with substantial opportunities for investors and innovators willing to engage at the intersection of computation, physics, and applied technology.
Future Outlook: Next-Gen Modeling, Collaboration, and Disruptive Potential
As the landscape of particle physics continues to evolve in 2025, quark exchange kinetics modeling remains at the frontier of both theoretical and computational research. This modeling approach, which focuses on the dynamic processes underlying quark interactions and exchanges within hadrons, is expected to see significant advances in the coming years, fueled by next-generation computational resources, collaborative frameworks, and cross-disciplinary innovation.
A major driver of progress in this field is the increasing integration of high-performance computing (HPC) and artificial intelligence (AI) into quantum chromodynamics (QCD) simulations. Institutions such as CERN and Brookhaven National Laboratory are deploying exascale computing platforms and advanced algorithms capable of handling the immense complexity of multi-quark systems. These tools are enabling more precise modeling of quark exchange rates, hadronization, and collective quark phenomena.
Collaborative efforts are also accelerating. International projects like the Lattice QCD collaborations are uniting resources and expertise from leading research centers, including Thomas Jefferson National Accelerator Facility and Oak Ridge National Laboratory. These collaborations are set to refine exchange kinetics models through more accurate lattice calculations and experimental validation, particularly as new data emerges from upgraded facilities such as the Large Hadron Collider and Electron-Ion Collider.
Looking ahead, disruptive potential lies in the convergence of quantum computing with quark kinetics modeling. Early-stage demonstrations by teams at IBM and Intel have shown that quantum processors could, within a few years, simulate aspects of QCD and quark exchange dynamics more efficiently than classical supercomputers. As quantum hardware matures, this could unlock real-time modeling of quark-gluon interactions, transforming our understanding of strong force phenomena.
Finally, open science initiatives are poised to democratize access to quark exchange models and data. Platforms promoted by industry bodies such as the Interactions Collaboration will likely foster broader participation and accelerate innovation through shared datasets, open-source simulation tools, and international hackathons.
In summary, the next few years will see quark exchange kinetics modeling propelled by unprecedented computing power, global scientific collaboration, and the early adoption of quantum technologies—setting the stage for breakthroughs that could reshape both theoretical physics and its technological spin-offs.
Sources & References
- IBM
- CERN
- Brookhaven National Laboratory
- NVIDIA
- Fermi National Accelerator Laboratory
- CERN
- CERN
- Fermi National Accelerator Laboratory
- NVIDIA Corporation
- IBM
- Deutsches Elektronen-Synchrotron (DESY)
- Lawrence Livermore National Laboratory
- Thomas Jefferson National Accelerator Facility
- CERN
- Oak Ridge National Laboratory
- RIKEN
- IEEE
- Interactions Collaboration