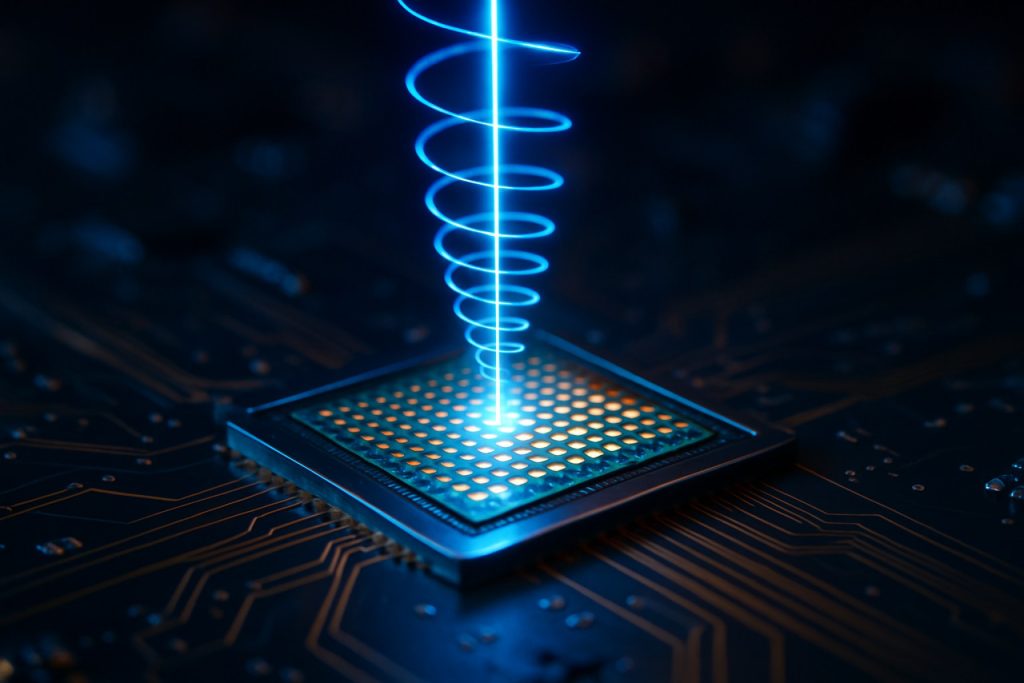
Spin-Based Nanophotonics in 2025: Unleashing Quantum Control for Ultra-Fast, Energy-Efficient Photonic Technologies. Explore How Spin Dynamics Are Shaping the Future of Optical Innovation.
- Executive Summary: Key Trends and Market Outlook (2025–2030)
- Technology Fundamentals: Spintronics Meets Nanophotonics
- Market Size, Segmentation, and Growth Forecasts
- Emerging Applications: Quantum Computing, Sensing, and Communications
- Key Players and Strategic Partnerships (e.g., imec-int.com, ibm.com, ieee.org)
- Materials Innovation: 2D Materials, Metasurfaces, and Hybrid Platforms
- Manufacturing Challenges and Scalability
- Regulatory Landscape and Standardization Efforts (e.g., ieee.org)
- Investment, Funding, and M&A Activity
- Future Outlook: Disruptive Potential and Roadmap to Commercialization
- Sources & References
Executive Summary: Key Trends and Market Outlook (2025–2030)
Spin-based nanophotonics is rapidly emerging as a transformative field at the intersection of photonics, quantum information science, and spintronics. As of 2025, the sector is witnessing accelerated research and early-stage commercialization, driven by the need for faster, more energy-efficient data processing and secure quantum communication. The core innovation lies in manipulating the spin of electrons and photons at the nanoscale, enabling new device architectures that surpass the limitations of conventional photonics and electronics.
Key trends shaping the market include the integration of spintronic materials—such as transition metal dichalcogenides and topological insulators—into photonic circuits, and the development of spin-based light sources, detectors, and modulators. Leading research institutions and technology companies are collaborating to translate laboratory breakthroughs into scalable components. For example, IBM is actively exploring spin-photon interfaces for quantum networks, while Intel is investing in spin-based optoelectronic integration for next-generation computing platforms. Additionally, National Institute of Standards and Technology (NIST) is standardizing measurement techniques for spin-photon interactions, which is crucial for industry-wide adoption.
In 2025, the market is characterized by pilot projects and prototype demonstrations, particularly in quantum communication and sensing. Spin-based single-photon sources and detectors are being tested for secure data transmission and ultra-sensitive magnetic field detection. The demand for these components is expected to grow as quantum networks and advanced sensing applications move toward commercialization. Companies such as Toshiba and Hitachi are expanding their quantum technology portfolios to include spin-based photonic devices, aiming to capture early market share in quantum-secure communications.
Looking ahead to 2030, the outlook for spin-based nanophotonics is robust, with anticipated compound annual growth rates in double digits as enabling technologies mature. The convergence of spintronics and photonics is expected to yield breakthroughs in on-chip quantum information processing, low-power optical interconnects, and novel sensors. Strategic partnerships between semiconductor manufacturers, quantum technology firms, and research organizations will be pivotal in overcoming fabrication and scalability challenges. As standardization progresses and pilot deployments prove viability, spin-based nanophotonics is poised to become a foundational technology for quantum-era information systems and advanced photonic devices.
Technology Fundamentals: Spintronics Meets Nanophotonics
Spin-based nanophotonics represents a convergence of spintronics and nanophotonics, leveraging the quantum property of electron spin to manipulate light at the nanoscale. This interdisciplinary field is rapidly evolving, with 2025 marking a period of intensified research and early-stage commercialization. The core principle involves controlling the spin angular momentum of photons and electrons to enable new functionalities in photonic devices, such as ultrafast data processing, low-power optical switches, and highly sensitive sensors.
Recent years have seen significant advances in the integration of magnetic materials with photonic structures. For instance, the use of two-dimensional (2D) materials like transition metal dichalcogenides (TMDs) and magnetic van der Waals crystals has enabled the demonstration of room-temperature spin-polarized light emission and detection. These breakthroughs are paving the way for practical spin-based light sources and detectors, which are essential for next-generation optical communication and quantum information systems.
Key industry players are actively developing spintronic and nanophotonic components. IBM has a longstanding research program in spintronics and quantum photonics, focusing on integrating spin-based logic with photonic circuits for scalable quantum computing architectures. Intel Corporation is exploring spin-based optoelectronic devices as part of its broader push into silicon photonics, aiming to enhance data transfer speeds and energy efficiency in data centers. Hitachi High-Tech Corporation is also investing in advanced nanofabrication tools that enable the precise patterning of spintronic-photonic hybrid devices.
On the materials front, Samsung Electronics is investigating the use of chiral nanostructures and magnetic semiconductors to achieve robust spin control in photonic circuits, with potential applications in secure communications and neuromorphic computing. Meanwhile, Toshiba Corporation is advancing quantum dot and spin-photon interface technologies, targeting quantum cryptography and ultra-sensitive imaging.
Looking ahead to the next few years, the outlook for spin-based nanophotonics is promising. The field is expected to benefit from continued miniaturization, improved material synthesis, and the development of scalable fabrication techniques. Industry collaborations and public-private partnerships are likely to accelerate the transition from laboratory demonstrations to commercial products. By 2027, early adoption in quantum communication networks, high-speed optical interconnects, and advanced sensing platforms is anticipated, positioning spin-based nanophotonics as a foundational technology for the photonic and quantum industries.
Market Size, Segmentation, and Growth Forecasts
Spin-based nanophotonics, an emerging field at the intersection of spintronics and photonics, is gaining momentum as researchers and industry players seek to exploit the spin degree of freedom of electrons and photons for next-generation information processing, sensing, and communication technologies. As of 2025, the market for spin-based nanophotonics remains in its nascent stage, primarily driven by R&D investments and early-stage commercialization in sectors such as quantum computing, secure communications, and advanced optical components.
The market size for spin-based nanophotonics is difficult to quantify precisely due to its overlap with broader nanophotonics and spintronics markets. However, the global nanophotonics market is projected to surpass USD 30 billion by 2025, with spin-based technologies expected to capture a growing share as prototype devices transition toward commercial viability. Key segmentation within the spin-based nanophotonics market includes:
- Device Type: Spin lasers, spin-LEDs, spin-based modulators, and non-reciprocal optical components.
- Application: Quantum information processing, optical interconnects, secure communications, and high-sensitivity sensing.
- End-User: Research institutions, semiconductor manufacturers, telecommunications, and defense sectors.
Several leading companies and research organizations are actively developing spin-based nanophotonic technologies. IBM is advancing spin-photon interfaces for quantum networks, while Intel and Samsung Electronics are exploring spintronic-photonic integration for future chip architectures. National Institute of Standards and Technology (NIST) is also supporting foundational research in spin-based photonic devices, particularly for quantum metrology and secure communications.
Growth forecasts for the next few years (2025–2028) indicate a compound annual growth rate (CAGR) in the high single digits for spin-based nanophotonics, outpacing the broader photonics sector due to increasing demand for quantum and spin-enabled functionalities. The market is expected to benefit from:
- Rising investments in quantum technologies and secure communication infrastructure.
- Collaborations between academia and industry to accelerate device prototyping and standardization.
- Government funding initiatives in the US, EU, and Asia-Pacific targeting quantum and spintronic research.
While commercial adoption is still limited, the outlook for spin-based nanophotonics is promising, with pilot deployments anticipated in quantum communication testbeds and advanced photonic circuits by 2027–2028. The sector’s growth will depend on continued advances in material science, scalable fabrication, and integration with existing semiconductor platforms.
Emerging Applications: Quantum Computing, Sensing, and Communications
Spin-based nanophotonics is rapidly advancing as a foundational technology for next-generation quantum computing, sensing, and secure communications. In 2025, the field is witnessing significant momentum, driven by breakthroughs in manipulating and detecting electron and nuclear spins at the nanoscale using photonic structures. These advances are enabling new device architectures that leverage the quantum properties of spins for practical applications.
A key area of progress is the integration of spin qubits—such as nitrogen-vacancy (NV) centers in diamond and silicon carbide—with photonic circuits. This integration allows for efficient spin-photon interfaces, which are essential for scalable quantum networks. Companies like Element Six, a subsidiary of the De Beers Group, are at the forefront of producing high-purity diamond substrates with engineered NV centers, supporting both academic and industrial research into quantum photonics. Similarly, Qnami is commercializing quantum sensors based on NV centers for nanoscale magnetic imaging, with applications in materials science and biology.
In quantum computing, spin-based nanophotonics is enabling the development of distributed quantum processors, where information is encoded in spin states and transmitted via single photons. This approach is being explored by organizations such as IBM and Intel, both of which are investing in spin qubit research and photonic interconnects to overcome the scaling limitations of traditional superconducting qubits. The ability to entangle distant spin qubits through photonic links is a critical milestone for building large-scale, fault-tolerant quantum computers.
Quantum sensing is another promising application, with spin-based nanophotonic devices offering unprecedented sensitivity to magnetic and electric fields, temperature, and strain at the nanoscale. These sensors are being deployed in diverse environments, from semiconductor wafer inspection to biological imaging. Qnami and Element Six are actively supplying components and turnkey solutions for these markets, and further product launches are anticipated in the next few years as device integration and robustness improve.
In quantum communications, spin-photon interfaces are central to the realization of quantum repeaters and secure quantum key distribution (QKD) networks. Efforts by Toshiba and ID Quantique are focused on developing practical QKD systems, with ongoing research into spin-based emitters and detectors to enhance performance and scalability.
Looking ahead, the next few years are expected to bring further convergence between spintronics and nanophotonics, with increased commercialization of spin-based quantum devices. As fabrication techniques mature and integration challenges are addressed, spin-based nanophotonics is poised to play a pivotal role in the quantum technology ecosystem, enabling new capabilities in computation, sensing, and secure communications.
Key Players and Strategic Partnerships (e.g., imec-int.com, ibm.com, ieee.org)
The landscape of spin-based nanophotonics in 2025 is shaped by a dynamic interplay of leading research institutes, technology companies, and strategic alliances. This field, which leverages the spin degree of freedom of electrons and photons for advanced photonic functionalities, is witnessing accelerated innovation due to collaborations between academia, industry, and standardization bodies.
A central player is imec, the Belgium-based nanoelectronics research hub. Imec’s extensive work in spintronics and photonics integration, particularly through its open innovation model, has enabled partnerships with global semiconductor manufacturers and photonics startups. Their pilot lines and prototyping services are crucial for translating spin-based nanophotonic concepts into scalable devices, with recent projects focusing on spin-controlled light sources and detectors for quantum and neuromorphic computing.
In the United States, IBM continues to be a frontrunner, leveraging its legacy in quantum information science and materials engineering. IBM’s research division is actively developing spin-photon interfaces and hybrid quantum systems, aiming to bridge the gap between spintronic memory and photonic interconnects. Their collaborations with universities and national labs are expected to yield demonstrators of spin-based photonic circuits within the next few years, targeting applications in secure communications and high-speed data processing.
Standardization and knowledge dissemination are driven by organizations such as the IEEE. The IEEE Photonics Society and Magnetics Society are facilitating the formation of working groups and technical committees dedicated to spin-based photonics, fostering interoperability and best practices. These efforts are critical as the field matures and moves toward commercial deployment, ensuring that device architectures and measurement protocols are harmonized across the industry.
Other notable contributors include NIST (National Institute of Standards and Technology), which is developing metrology tools for characterizing spin-photon interactions at the nanoscale, and Hitachi, which is exploring spin-based photonic devices for next-generation data storage and optical computing. European consortia, often coordinated by CORDIS under the Horizon Europe framework, are also fostering cross-border partnerships, pooling expertise in materials science, device engineering, and system integration.
Looking ahead, the next few years are expected to see intensified collaboration between these key players, with joint ventures and public-private partnerships accelerating the path from laboratory breakthroughs to market-ready spin-based nanophotonic technologies.
Materials Innovation: 2D Materials, Metasurfaces, and Hybrid Platforms
Spin-based nanophotonics is rapidly advancing, driven by innovations in materials science, particularly in the development and integration of 2D materials, metasurfaces, and hybrid platforms. As of 2025, the field is witnessing significant momentum due to the unique ability of these materials to manipulate the spin degree of freedom of photons, enabling new paradigms in information processing, quantum communication, and sensing.
Two-dimensional (2D) materials, such as transition metal dichalcogenides (TMDs) and hexagonal boron nitride (hBN), are at the forefront of this revolution. These atomically thin materials exhibit strong spin-orbit coupling and valley-selective optical transitions, making them ideal for spin-photon interfaces. Companies like Graphenea and 2D Semiconductors are actively supplying high-quality 2D crystals and heterostructures, supporting both academic and industrial research into spin-based photonic devices. The integration of these materials with photonic circuits is expected to accelerate, with scalable wafer-level production techniques under development to meet the demands of quantum and classical photonic applications.
Metasurfaces—engineered arrays of subwavelength nanostructures—are another key enabler for spin-based nanophotonics. By precisely controlling the local polarization and phase of light, metasurfaces can generate and manipulate spin-dependent optical phenomena such as the photonic spin Hall effect and chiral light-matter interactions. Leading manufacturers like Metamaterial Inc. and META are commercializing metasurface technologies for applications ranging from advanced displays to quantum optics. In 2025, the focus is on integrating metasurfaces with active materials and tunable platforms, enabling dynamic control over spin-polarized light at the nanoscale.
Hybrid platforms that combine 2D materials, metasurfaces, and conventional photonic components are emerging as a promising route to scalable, multifunctional spin-photonic devices. These platforms leverage the strengths of each material system, such as the strong light-matter interaction of 2D materials and the versatile wavefront shaping of metasurfaces. Collaborative efforts between material suppliers, device manufacturers, and research institutions are expected to yield prototype devices for spin-based quantum information processing and secure communication within the next few years.
Looking ahead, the outlook for spin-based nanophotonics is robust. The convergence of advanced materials, scalable fabrication, and device integration is poised to unlock new functionalities in photonic chips, sensors, and quantum networks. As industry players like Graphenea, 2D Semiconductors, and Metamaterial Inc. continue to expand their capabilities, the commercialization of spin-based nanophotonic technologies is expected to accelerate, with early adoption in quantum communication and next-generation optoelectronic systems anticipated by the late 2020s.
Manufacturing Challenges and Scalability
Spin-based nanophotonics, which leverages the spin degree of freedom of photons and electrons for information processing and transmission at the nanoscale, is rapidly advancing toward practical applications. However, manufacturing challenges and scalability remain significant hurdles as the field moves into 2025 and the near future.
A primary challenge lies in the precise fabrication of nanostructures that can manipulate spin states with high fidelity. Techniques such as electron-beam lithography and focused ion beam milling are widely used for prototyping, but their throughput and cost are prohibitive for large-scale production. Efforts to transition to scalable methods, such as nanoimprint lithography and advanced photolithography, are ongoing. For example, ASML, a global leader in photolithography systems, is actively developing next-generation extreme ultraviolet (EUV) lithography tools that could enable the mass production of nanophotonic devices with sub-10 nm features, a critical requirement for spin-based architectures.
Material quality and integration also present substantial obstacles. Spin-based nanophotonic devices often require materials with long spin coherence times and low defect densities, such as high-purity diamond for nitrogen-vacancy (NV) centers or transition metal dichalcogenides (TMDs) for valleytronic applications. Companies like Element Six are scaling up the production of synthetic diamond substrates with controlled defect profiles, which are essential for reproducible device performance. Meanwhile, Oxford Instruments provides advanced deposition and etching systems tailored for the fabrication of 2D materials and heterostructures, supporting the integration of spintronic and photonic functionalities.
Another key issue is the alignment and coupling of spin-based nanophotonic elements with conventional photonic and electronic circuits. Achieving high-yield, wafer-scale integration without degrading spin properties is a nontrivial task. Industry consortia and research alliances, such as those coordinated by imec, are working to develop standardized process flows and hybrid integration techniques that bridge the gap between laboratory demonstrations and manufacturable systems.
Looking ahead, the outlook for scalable manufacturing of spin-based nanophotonic devices is cautiously optimistic. The convergence of advanced lithography, high-quality material synthesis, and hybrid integration platforms is expected to enable pilot production lines by the late 2020s. However, widespread commercialization will depend on further improvements in yield, reproducibility, and cost-effectiveness, as well as the establishment of industry-wide standards for device performance and reliability.
Regulatory Landscape and Standardization Efforts (e.g., ieee.org)
The regulatory landscape and standardization efforts for spin-based nanophotonics are evolving in tandem with rapid technological advancements in the field. As of 2025, the sector is characterized by a growing need for harmonized standards to ensure interoperability, safety, and reliability of devices leveraging spintronic and photonic phenomena at the nanoscale. Spin-based nanophotonics, which exploits the spin degree of freedom of electrons and photons for information processing and communication, is increasingly intersecting with quantum technologies, optoelectronics, and advanced materials, prompting regulatory bodies and industry consortia to address emerging challenges.
The IEEE has been at the forefront of standardization in photonics and spintronics, with several working groups focusing on quantum devices, nanophotonic components, and spin-based information processing. In 2024 and 2025, IEEE’s Nanotechnology Council and Photonics Society have initiated discussions on frameworks for device characterization, measurement protocols, and data formats specific to spin-based nanophotonic systems. These efforts aim to facilitate compatibility across manufacturers and research institutions, and to accelerate commercialization by reducing technical barriers.
In parallel, international bodies such as the International Electrotechnical Commission (IEC) and the International Organization for Standardization (ISO) are monitoring developments in nanophotonics and quantum technologies. While no dedicated standards for spin-based nanophotonics have been published as of early 2025, both organizations have active technical committees (e.g., IEC TC 113 for nanotechnology standardization) that are expected to address spintronic-photonic integration as the technology matures.
Industry stakeholders, including leading component manufacturers and research-driven companies, are increasingly participating in pre-standardization activities. For example, IBM and Intel—both with significant investments in spintronics and nanophotonics R&D—are contributing to collaborative consortia and public-private partnerships aimed at defining best practices for device fabrication, testing, and system integration. These companies are also engaging with regulatory agencies to ensure that emerging standards reflect real-world manufacturing and operational requirements.
Looking ahead, the next few years are expected to see the publication of foundational guidelines and technical specifications for spin-based nanophotonic devices, particularly as applications in quantum communication, sensing, and computing move closer to commercialization. Regulatory focus will likely intensify on issues such as electromagnetic compatibility, device reliability, and environmental safety, with harmonization across regions being a key priority. The ongoing collaboration between industry, academia, and standards organizations is poised to shape a robust regulatory framework that supports innovation while safeguarding users and the broader ecosystem.
Investment, Funding, and M&A Activity
Investment and funding activity in spin-based nanophotonics has accelerated in 2025, driven by the convergence of quantum information science, photonic integration, and the demand for energy-efficient data processing. The sector, which leverages the spin degree of freedom of electrons and photons for manipulating light at the nanoscale, is attracting both public and private capital, with a focus on commercializing spintronic-photonic devices for quantum computing, secure communications, and advanced sensing.
Several leading photonics and semiconductor companies have increased their strategic investments in spin-based nanophotonics. IBM continues to expand its quantum and nanophotonics research, with recent funding rounds supporting collaborative projects with academic institutions and startups focused on spin-photon interfaces. Intel Corporation has also announced new investments in spintronic materials and integrated photonic platforms, aiming to enhance the scalability and efficiency of quantum and neuromorphic computing architectures.
On the startup front, venture capital interest is robust. Companies such as Quantinuum and PsiQuantum—both recognized for their quantum photonics expertise—have secured additional funding rounds in 2024–2025, with a portion earmarked for research into spin-based photonic components. These investments are often supported by government innovation programs in the US, EU, and Asia, reflecting the strategic importance of spin-based nanophotonics for next-generation information technologies.
Mergers and acquisitions are also shaping the landscape. In early 2025, Infineon Technologies AG completed the acquisition of a European spintronics startup specializing in spin-based light modulators, aiming to integrate these components into its photonic chip portfolio. Meanwhile, NXP Semiconductors has entered into a joint venture with a leading research institute to accelerate the commercialization of spin-photonics for secure communications and automotive LiDAR.
Looking ahead, the outlook for investment and M&A in spin-based nanophotonics remains strong. The sector is expected to see continued inflows as device performance improves and pilot applications in quantum networks and photonic computing move closer to market. Strategic partnerships between established semiconductor manufacturers and innovative startups will likely intensify, with a focus on scaling up fabrication and integrating spin-based photonic devices into mainstream technology platforms.
Future Outlook: Disruptive Potential and Roadmap to Commercialization
Spin-based nanophotonics, which leverages the quantum property of electron spin to manipulate light at the nanoscale, is poised for significant advances in 2025 and the following years. This field is at the intersection of photonics, quantum information science, and materials engineering, with the potential to disrupt conventional photonic and electronic technologies by enabling ultra-compact, energy-efficient, and high-speed devices.
In 2025, the focus remains on overcoming key technical challenges such as room-temperature operation, scalable fabrication of spintronic-photonic devices, and integration with existing semiconductor platforms. Major research institutions and industry players are intensifying efforts to develop spin-based light sources, modulators, and detectors that can be seamlessly incorporated into photonic integrated circuits. For example, IBM continues to invest in quantum and spintronics research, aiming to bridge the gap between laboratory demonstrations and practical, manufacturable devices. Similarly, Intel is exploring spin-based approaches for next-generation data interconnects and logic, with a focus on compatibility with CMOS processes.
Material innovation is a critical driver. The development of two-dimensional materials such as transition metal dichalcogenides (TMDs) and topological insulators, which exhibit strong spin-orbit coupling and robust spin coherence, is accelerating. Companies like Oxford Instruments are supplying advanced deposition and characterization tools to enable precise engineering of these materials at the atomic scale. Meanwhile, Nanoscribe is providing high-resolution 3D nanofabrication systems that are essential for prototyping complex spin-photonic architectures.
The roadmap to commercialization involves several stages. In the near term (2025–2027), expect to see demonstration of spin-based nanophotonic components in niche applications such as quantum communication, secure data links, and specialized sensors. Collaborative projects between academia and industry, often supported by government initiatives, are expected to yield prototype devices with improved performance metrics—such as lower energy consumption and higher data rates—compared to traditional photonic components.
Looking further ahead, the integration of spin-based nanophotonics with mainstream silicon photonics platforms is anticipated to unlock broader markets, including data centers, telecommunications, and advanced computing. Standardization efforts, led by industry consortia and organizations such as SEMI, will be crucial for ensuring interoperability and accelerating adoption. As fabrication techniques mature and costs decrease, spin-based nanophotonics could become a foundational technology for the next generation of information processing and communication systems.
Sources & References
- IBM
- National Institute of Standards and Technology (NIST)
- Toshiba
- Hitachi
- Qnami
- ID Quantique
- imec
- IEEE
- CORDIS
- 2D Semiconductors
- Metamaterial Inc.
- META
- ASML
- Oxford Instruments
- International Organization for Standardization (ISO)
- Quantinuum
- Infineon Technologies AG
- NXP Semiconductors
- Nanoscribe